Meet Ten of the Next Five Successful Advanced Reactors
These are the advanced systems that are closest to deployment
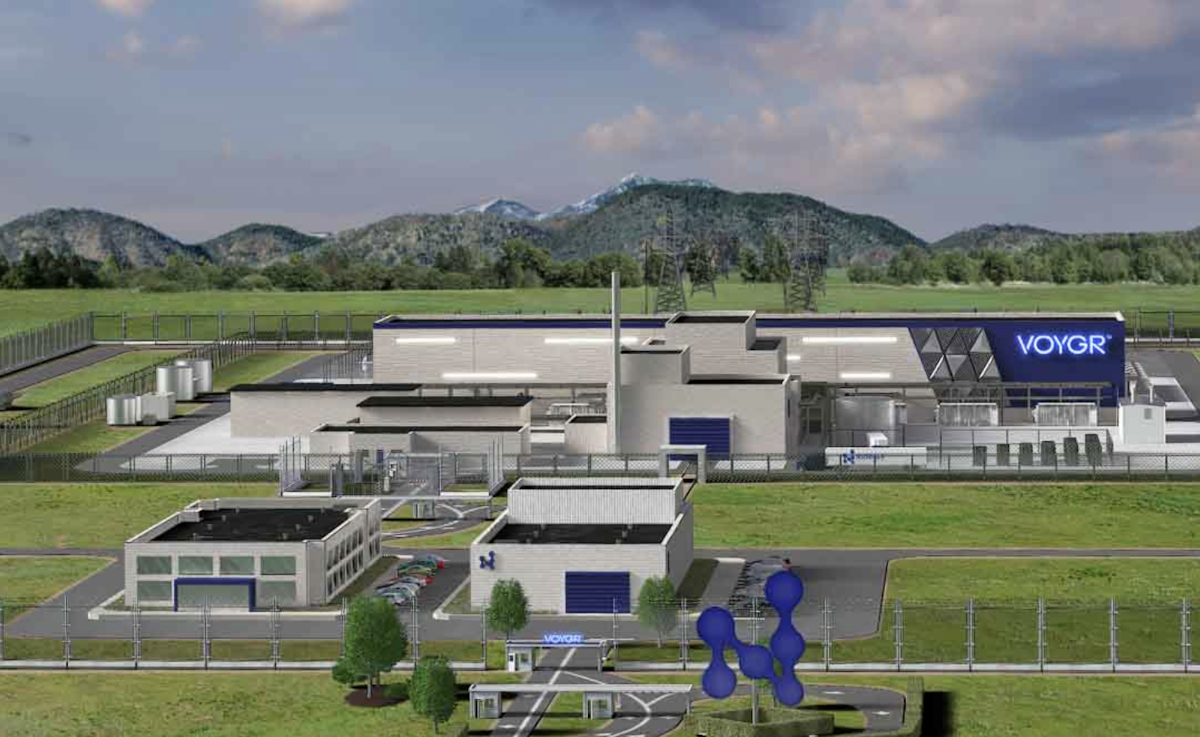
-
-
Share
-
Share via Twitter -
Share via Facebook -
Share via Email
-
American inventors and engineers have lately responded to the challenge of cleaning up the energy system the way they always do when faced with an urgent task: with a swarm of new ideas, some of which can be put into service quickly, some in the longer term, and some probably never.
That also goes for the glut of new reactor designs now under development.
It’s hard to tell now which designs will turn into commercial successes—soon or in ten or fifteen years—which will find at least a niche application, and which will follow the paths of cellulosic ethanol or nanotechnology, to name two much-hyped ideas from decades ago that have not lived up to the hopes of developers. And all of them face challenges, including a rigid and hyper-conservative Nuclear Regulatory Commission, and the reluctance of utility companies to be the first to try out a new technology.
But it is possible to at least categorize the reactors now under development into those with near-term prospects (that is, a good shot at success in the 2030s) and those that are probably further down the line, if they mature at all.
First, it is worth noting that there is no hard-and-fast definition of an “advanced” reactor. Some of the next generation reactors make smart, evolutionary changes to existing designs. Others are radical departures from today’s fleet. Generally speaking, the degree of innovation and nearness to commercialization are inverse.
Second, all reactors—today’s and tomorrow’s—can be categorized by the coolant and/or moderator they use. The coolant is the liquid or gas that is heated by the nuclear reaction, and which carries that heat away from the reaction to where it can be turned into steam, spin a turbine to make electricity, or do other kinds of work. The moderator is the material that slows down the neutrons that are emitted when an atom is split, to a speed most likely to cause another fission reaction, and sustain a nuclear chain reaction. In the United States, all the power reactors now running use ordinary water (called “light water”) as both the coolant and the moderator. In some designs now being readied for commercial use, the coolant is molten salt, molten metal, or an inert gas. And some of the new designs do not use a moderator, but operate on fast neutrons (and are known as “fast reactors”).
At the moment, the new reactors with the shortest path to commercial deployment look to be light-water reactors: they use fuel that is already commercially produced and most of their various components are already in production. Of the new light-water reactors, the three that seem most likely to be commercialized first (detailed below) are all “small modular reactors,” meaning that they can be factory-manufactured, and built faster, allowing for lower costs and easier quality control. They are also designed with far simpler emergency cooling systems than today’s fleet, reducing their size, complexity, and cost. The trade-off is that these machines are mostly designed to make electricity, although they may also be used to desalinate seawater.

Light Water
NuScale Power
NuScale, a start-up that has signed up many industrial companies as supplier/investors, has won a license from the NRC for clusters of small modular reactors. The modules, 77 megawatts each, are mounted in a design suggesting a Thermos bottle. Each reactor sits in metal cylinder, immersed in a giant pool of water. The reactor is separated from the walls of the cylinder by a vacuum layer. If the regular cooling system fails, the vacuum is broken and excess steam condenses inside that insulating layer. Heat is conducted passively through the reactor vessel, through the condensed water, and then to the cylinder, and from there to the giant pool. The reactor stays safe even without electricity, or pumps, or operator actions.
It runs on standard Westinghouse-type fuel, in shorter bundles than conventional reactors.
A coalition of public power entities in the western U.S. states has been negotiating to build a NuScale plant at the Idaho National Laboratory; the company also has prospects in Eastern Europe.Learn more in Nuclear Notes.
BWRX-300
GE-Hitachi has designed a tenth version of the boiling water reactor. (Hence the Roman numeral for ten in the title.) The design draws heavily on earlier boiling water reactors, and 80 percent of the components are already in use in the nuclear industry, according to the company. Smaller size makes the emergency core cooling system much simpler.
Ontario Power Generation has broken ground to prepare a site for a BWRX, and theTennessee Valley Authority has strong interest. Learn more in Nuclear Notes.
AP300
Westinghouse’s “Advanced Passive” design is in service in China, and is nearing completion at Plant Vogtle in Georgia, in an 1,100 megawatt version. Compared to older Westinghouse designs, it has vastly reduced requirements for safety-grade pumps, valves, and piping. Now it is offering a small modular reactor version, at 300 megawatts. Like GE-Hitachi, Westinghouse says that it is reusing numerous components that are already in service elsewhere, and has a good start on the work needed to get a license from the Nuclear Regulatory Commission.

After light-water reactors, the next type of reactor likely to come online are two designs that are more ambitious and more capable. One runs at higher temperatures, which means it can do more kinds of work; the other incorporates a thermal storage battery and has greater fuel flexibility.
In technology, they do not have anything in common with each other. But they are each in the first tranche of projects supported by the Energy Department’s Advanced Reactor Demonstration Program. That program, administered by the Energy Department, provides a dollar-for-dollar match for the cost of the first reactor, up to $1.6 billion for each reactor. It is supposed to produce a working model in five to seven years of the grant award, which was in 2020. But this seems unlikely. Learn more in Nuclear Notes.
What puts these reactors ahead of some others is that, while sketching out a new design is fairly easy, developing the detail needed to apply for a license and begin construction is much more expensive. And these reactors have strong financial backing from the Department of Energy.

Advanced Reactor Flagship Projects
Natrium
The project is a combination fast reactor and thermal battery, and is designed to rescue the grid from the daily swings in solar energy production. The reactor gives a steady output of enough heat to generate 345 megawatts, but that heat does not go directly to make steam, as happens in the reactors in service today. Rather, it heats up a big tank of molten salt. On the other side of the salt tank, heat can be withdrawn to make steam and spin turbines. The turbines can vary from 100 megawatts to 500 megawatts.
The molten salt storage means that output from the plant can easily be ramped up and down. At noon, when solar is at its peak, for example, the plant can send out to 100 megawatts even as the reactor continues to run at 500 megawatts. When the sun starts to go down, when people are arriving home from work, plugging in their electric cars, cranking up the home air conditioning, running their microwaves, and big-screen tvs, the Natrium plant can start discharging its battery.
The reactor has no moderator, so the neutrons liberated by splitting uranium atoms are flying around at an appreciable fraction of the speed of light. With that extra energy, they can split atoms that won’t split in today’s reactors.
For example, they can split U-238, the dominant form of uranium on earth, of which we have vast quantities. They can split some of what we now call nuclear waste, atoms that are only slightly radioactive, but will remain radioactive for millennia, and thus become more difficult to dispose of. If those atoms, which are fragments of uranium, are split again, they will give off more energy. And they will, paradoxically, become MORE radioactive. That’s good, because they’ll burn out faster. That makes for easier disposal.
There are two factors, though, that will prevent Natrium technology from arriving immediately. First, it needs uranium at an enrichment of approximately 1 part 235 to four parts 238. The highest enrichment now commercially available is 1 part 235 to 19 parts 238. The original plan was to buy that high-enriched fuel from a government-owned company in Russia, but with the war in Ukraine, that option is off the table.
The reactor still does have an advantage in simplicity of construction. Sodium can be heated very hot and remain at low pressure, unlike water, which in today’s reactors attains a pressure of up to 2,200 pounds per square inch. A potential problem is the NRC’s very limited experience in regulating reactors cooled by anything other than light water. The company is engaged in various pre-application activities with the NRC, like submitting plans for how to qualify its fuel.
The effort is a partnership between GE-Hitachi and a company founded by Bill Gates, who repeatedly stresses that nuclear energy can scale up to meet the global climate challenge. Learn more in Nuclear Notes.
Xe-10
X-energy’s entry is moderated by solid graphite and cooled by an inert gas, helium. This combination allows very high heat at only modest pressures, and makes the reactor suitable for very efficient electricity production (with fewer units of heat needed for each kilowatt-hour generated) and for high-temperature steam used in industrial applications (which is currently generated by burning fossil gas).
The fuel is uranium or other fissile materials encapsulated in layers of ceramic, in a form called “pebble bed.” In fact, the pebbles are closer to ping pong balls or billiard balls. The pebbles are swapped out while the reactor is running, like a gumball machine with a child putting quarters in at the outflow, as a technician re-loads from the top.
The first customer is Dow, the chemical company, at a plant in Seadrift, Texas. Dow is accustomed to big projects, and has extensive in-house engineering resources. And it is in a business where it can get a competitive edge by saying that its products have a smaller carbon footprint than its competitors’ products. As with Natrium, the company is engaged in various pre-application activities with the NRC.
But the Xe-100, like Natrium, needs higher enrichment fuel, which may be the factor that sets the date that it can be deployed. Learn more in Nuclear Notes.

After this category of reactor comes five projects that can broadly be seen as “risk reduction” efforts. This includes five other designs for plants supported by the Energy Department with the aim to get a clear, detailed idea of what it would take to build them. Commercial deployment is anticipated in ten to 14 years, i.e., five to seven years behind the flagship projects. But some of the very small reactors have faster timelines.

“Risk Reduction” Projects
KP-FHR
Kairos Power’s technology uses ingredients similar to what other designers are employing, but in a different combination. It is a pebble-bed reactor, with the fuel encapsulated in multiple layers of heat-resistant material, but the pebbles do not give off their heat to gas. Rather, they float in a molten mixture of fluoride salt. Kairos is highly unusual for planning to build a series of small test reactors. The first will not make electricity but will demonstrate the heart of the technology. The NRC recently approved the test reactor, called Hermes.
Molten salt reactors can operate at very high temperatures, but salt does not expand when heated the way water does, so they can run at low pressure, simplifying fabrication of the reactor and associated piping. And the higher heat can do many kinds of work beyond making electricity. (Although if all it does is make electricity, it will operate at very high efficiency, requiring less heat to make a kilowatt-hour than reactors that run at lower temperatures.)
But reactors that do not use ordinary water as their heat-transfer mechanism may require different materials, and different systems to handle the coolant and the fuel. Among the complications of using molten salt, inspecting the insides of vessels and piping can’t be done with a simple underwater camera.
None of these problems is impossible to solve, but they put these advanced reactors on a longer path to deployment. Learn more in Nuclear Notes.
SMR-160
Holtec, which has a highly successful business decommissioning old reactors and building dry casks for used nuclear fuel, and which builds heat exchangers for nuclear and non-nuclear plants, has designed a 160-megawatt Small Modular Reactor. Key components of that reactor would be built in its own manufacturing plant.
It has applied for a patent that would make the reactor particularly well-suited for a drop-in replacement for the boiler in a coal-fired power plant, although the reactor makes steam at lower temperature and lower pressure than a coal boiler. The Holtec system would compress the steam to resemble what a coal plant makes. At the plant, the turbine, generator, and other equipment would be re-used. It calls the reactor a “green boiler.”
eVinci
Westinghouse has designed a microreactor for use remote areas, or in places on the grid that need a very reliable electricity supply, or possibly the Moon. It is transportable, can be set up in less than 30 days, and also uses fuel in pebble form. Energy is removed from the core by “heat pipes,” and converted directly into electricity. Learn more in Nuclear Notes.
BWXT Advanced Nuclear Reactor (BANR)
The company, which has long experience making reactor fuel for the U.S. Navy, has designed a reactor that can be transported in standard shipping containers, by truck, ship, or rail, and will provide 50 megawatts of thermal energy, abut 17 megawatts of electricity. The fuel is also in pebble form but is different from what x-Energy and Kairos will use, and it produces more energy.
Molten Chloride Reactor Experiment
Southern Company, working with TerraPower (Bill Gates’ company) and three other organizations, is planning to build and operate a small “fast” reactor with no moderator. It could use a variety of fuels, including the spent fuel from conventional water-based reactors, because the higher-energy neutrons can split more kinds of atoms.
Russia operates two fast reactors, but these use molten sodium (a metal) as a coolant. Southern’s reactor would use molten salt, which makes them first-of-a-kind. And the salt would not only carry away the heat that the reactor produced; it would also carry the fuel, in dissolved form. The plan is to demonstrate a commercial model in the early 2030s. Among the many steps needed is manufacturing the salt. Learn more in Nuclear Notes.

Stepping back to view these competing designs as a group, it’s clear that advanced nuclear is on a path to a far larger diversity of products than exists in the fleet today. This shouldn’t be a surprise; all of today’s reactors were intended to produce baseload electricity, and tomorrow’s reactors will be required to do more, to decarbonize other sectors and to mesh well with a grid that is heavy with intermittent generators that depend on weather.
Just how many different roles advanced reactors will play is yet to be determined. And their success may depend on external factors, like the direction of the public consensus about what is needed to stabilize the climate. There will also be internal factors: Technical hurdles, some foreseen now, and some that are likely to be obvious only later.
For the time being, it may be sufficient to observe that in any technical field, the number of designs always exceeds the number of products that are successfully built. But designs that don’t get tried don’t get commercially deployed.

Other entrants
Oklo
Oklo, a Silicon Valley start-up, has designed a fast reactor called the Aurora Powerhouse, which is intended for remote, off-grid use. Oklo (the name refers to natural reactors that ran in Africa, millions of years ago, in rich uranium deposits) has plans for fast deployment, and has permission to build at the Energy Department’s vast Idaho National Laboratory. The department is also providing the fuel, left over from an earlier experiment. The reactor uses heat pipes to conduct energy from the core to where it can be converted directly into electricity. The reactor is underground, and in case of cooling system malfunction, excess heat can be conducted away through the surrounding rock. Learn more in Nuclear Notes.
But the Nuclear Regulatory Commission, after receiving Oklo’s application for a combined construction permit and operating license, rejected it in January 2022 as incomplete.
Moltex Stable Salt Reactor
Moltex uses molten fluoride salt at atmospheric pressure, making fabrication of the reactor and piping much simpler. The fuel is dissolved in salt, but contained in fuel assemblies. Salt also circulates outside the fuel assemblies, carrying away the heat. Fission products, if they escaped the assemblies, would be retained in the coolant salt.
The reactor can run on the spent fuel from other reactors of various types. The first model may be built adjacent to a CANDU reactor at Pt. Lepreau, in New Brunswick. The company has good support from the Canadian federal government, the province of New Brunswick, the U.S. Department of Energy and Ontario Power Generation.
