How Anomalous Science Breaks Through
An assessment of the current state and future of cold fusion research
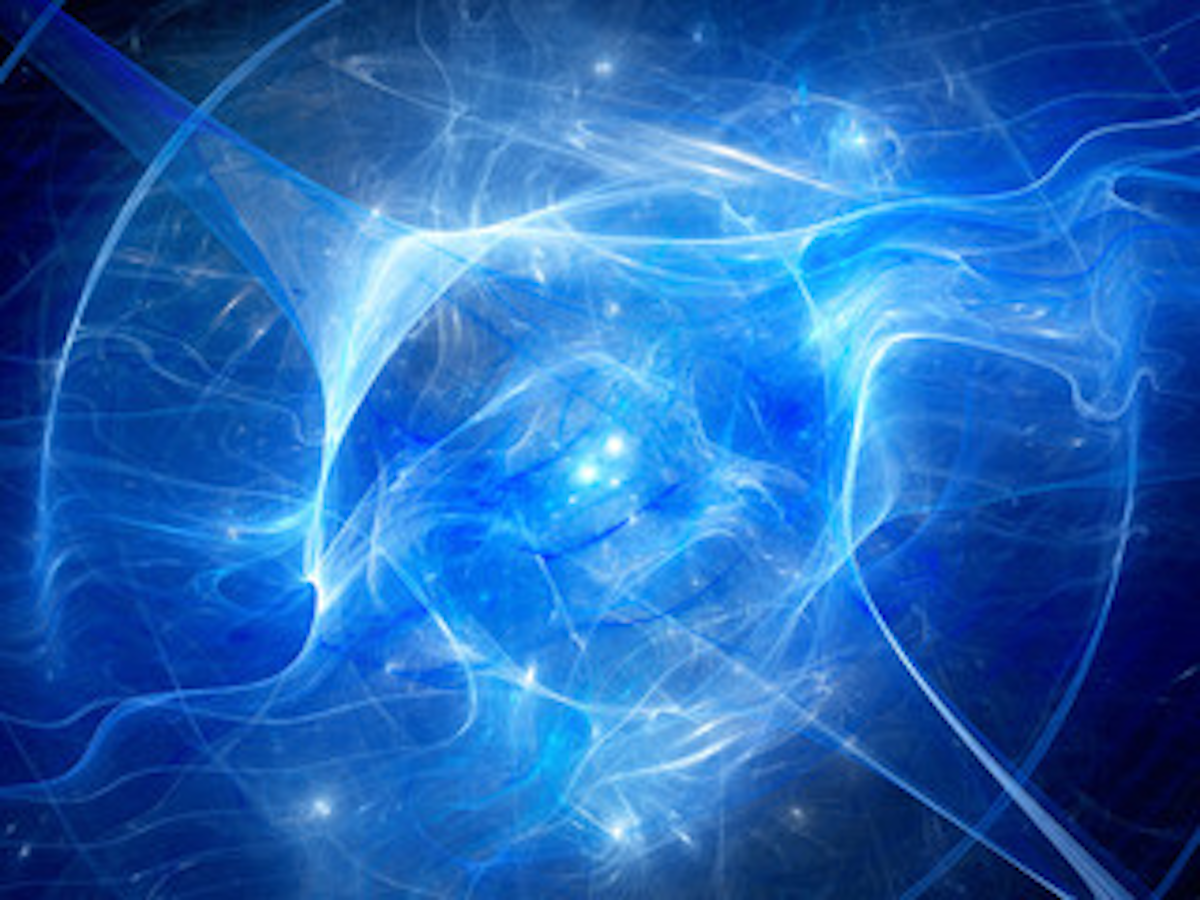
-
-
Share
-
Share via Twitter -
Share via Facebook -
Share via Email
-
Earlier this year I wrote “Fusion runs and cold.” The crux of the piece was that cold fusion, or low-energy nuclear reactions (LENR), has been wrongly excoriated from the mainstream institutions of science. And that there is intriguing experimental evidence for anomalous LENR phenomena and good theoretical motivation to pursue further work in this field. It is worth reading that article first, to contextualize this piece.
This summer, I attended the 25th International Conference on Condensed Matter Nuclear Science (ICCF) in Szczecin, Poland, my second conference in the ICCF series that started in 1990. The meeting featured a combination of stellar talks on exciting new research and eccentric presentations on anomalous phenomena likely inconsistent with LENR, but nevertheless curious.
What I have come to learn working in an admittedly fringe field is that the variance in the quality of research is high. But also that far-out, radical scientific exploration, albeit without access to all the modern tools that physical science has to offer, can be deeply valuable. As Dr. Florian Metzler put it, science has historically benefited from scouts that explore beyond the boundaries of conventional research, and present new ideas and preliminary findings for consideration by the community at large. Ultimately, such exploratory investigation requires the full suite of scientific methods and rigor to be brought to bear and – where applicable – to be integrated into the mainstream scientific fold.
LENR is a field without a reference experiment and still subject to both understandable, but respectful, skepticism as well as dismissive scorn and uncollegial mockery. Columbia political science Professor Andrew Gelman’s blog titled “Genius is not enough: The sad story of Peter Hagelstein, living monument to the sunk-cost fallacy” is a good example of the latter. In it, Gelman, presupposes that cold fusion is sunk and in place of technical arguments, he bludgeons MIT Professor Peter Hagelstein — a longtime cold fusion scientist and protege of Edward Teller, who first encouraged him to study the phenomena—to make his point. He is sure to point out that at the time of the notorious 1989 announcement of cold fusion, nobody was laughing because it was not considered bogus then, leaving the unwitting reader to safely assume, as the uninformed Gelman clearly has, that it is safely a laughable subject now. Gelman clarified via email correspondence that he has no objection to people working in cold fusion, but articles like his contribute to a culture that stigmatizes the field and its researchers without attempting to engage with the ideas at hand.
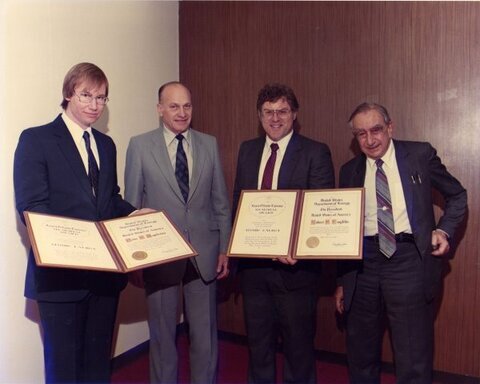
Dr. Peter Hagelstein (furthest left) awarded the 1984 E. O. Lawrence Nuclear Technology Award for his work in inventing the X-ray laser pictured with Lawrnece Livermore National Laboratory Direcotr Dr. Roger Bazel (second from the left), Stanford Professor Robert Laughlin (third from the left), and Dr. Edward Teller (fourth from the left).
All too often, cold fusion as a concept is incorrectly framed as violating physical laws. Some discussions of cold fusion, like this one with science celebrity Michio Kaku and science journalist Charles Seife, are collegial, but totally ignorant of experimental evidence far more compelling than the canonical excess heat reports of 1989 and relevant theoretical insights that go beyond mundane two-body quantum tunneling fusion rate calculations (e.g., collective coherent nuclear decay and reaction rate enhancement).
And then there is a contingent of the scientific community hell-bent on a self-righteous crusade to ridicule and ostracize research that is perceived to fall outside the mainstream, such as Robert Park in his book Voodoo Science and comments by Princeton physics professor — and notorious climate science skeptic – William Happer. There is a sort of cultural cache or social currency in science derived by signaling a performative and excessive skepticism to one's colleagues.
But the tone is changing as evidenced by the U.S. Department of Energy’s recent $10 million ARPA-E LENR research program — which kicked off in earnest last month — and by recent articles in Popular Science, the Financial Times, and IEEE Spectrum, podcasts, Google’s LENR research efforts, Defense Intelligence reports, and thoughtful science commentaries.
What LENR needs now, is a contemporary, well-characterized, and unambiguous replication of a reported nuclear anomaly — by which I mean an experimental result that is both clearly nuclear in origin and unexplained by conventional nuclear theory — published in a prominent journal by a highly credible research team. To be clear, there are already several high-quality nuclear anomalies reported in the existing LENR literature — some in mainstream publications, peer-reviewed journals and even a recent University of Illinois thesis, and others in the field’s Journal of Condensed Matter Nuclear Science — but they were either published too long ago, left important questions unaddressed, or have not been digested by the broader scientific community. By all accounts, the best chance for a phase change in the field is the ARPA-E LENR research program.
Emergent anomalous science
Low-energy nuclear reactions are an anomalous phenomenon. Because the underlying theory is not well understood and controlling variables such as precise nanostructures in the solid state is notoriously difficult, reproducing results in LENR has proved challenging. This is painfully not understood by science journalists, professional “debunkers,” and even Nobel Prize-winning scientists, all of whom have an idealized, ahistoric, and linear view of scientific discovery and progress.
All that said, longtime scientists and research groups in the field are, with increasing frequency, reporting excess heat, the observable most commonly associated with cold fusion. Excess heat describes the measured thermal output power that exceeds electrical input power to an electrolysis cell (conventionally a match of input and output power would be expected). The hypothesis is that excess heat is caused by the release of nuclear binding energy through low-energy nuclear reactions. But, as I have written before, and ARPA-E stressed in their funding opportunity announcement, such kind of evidence for LENR is insufficient due to the ambiguous nature of heat, numerous confounding variables, potential sources of measurement error, and possible prosaic explanations, not to mention that unless the excess heat is enormous, it does not immediately necessitate a nuclear origin (but for instance overlooked chemical reactions taking place in the sample). That said, in some reports of excess heat, the integrated excess thermal energy released is greater than that of possible prosaic chemical reactions. Moreover, the LENR field is well aware of possible confounding variables in measuring excess heat and the highest quality studies of excess heat have taken care to ensure accuracy.
But this is not the first time solid-state physics has produced anomalous phenomena with ambiguous origins and met with hefty skepticism from the broader scientific community.
The transistor — long thought to be simply a stroke of genius by Nobel Prize-winning physicists John Bardeen, William Shockley, and Walter Brattain of the highly esteemed Bell Labs — went through similar trials and tribulations in achieving reproducibility, elucidating theoretical mechanisms, and garnering mainstream acceptance. In 1948, these Bell Labs researchers published a Physics Review letter establishing the veracity of the transistor. However, anomaly reports of solid-state amplification effects in semiconductor crystals date to the early 1910s and the first patent for a solid-state crystal switch (i.e. transistor) was filed by Julius Edgar Lilienfeld as early as 1926. And yet the highly regarded Wolfgang Pauli, one of the progenitors of quantum physics, remarked “one shouldn't work on semiconductors, that is a filthy mess; who knows whether any semiconductors exist.”
Indeed, many today consider the solid-state nuclear anomalies in LENR over the past three and a half decades to be dubious. To be more precise, most scientists are likely unaware that any anomalies exist. But by the same token, LENR holds the promise to be the transistor of energy. ARPA-E called LENR “potentially the ideal form of nuclear energy.”
What then can the LENR field learn from the emergence of the transistor from a body of anomalies to a dominant MOS-FET transistor design and ultimately the integrated chips that prove so valuable to our world today?
If cold fusion is to break through
There are three core lessons for the LENR field to heed from the evolution of the transistor.
First, tacit knowledge gained by practitioners of an anomalous science is highly valuable. While deeper insights are limited by theoretical understanding of the mechanism at hand, experience in producing anomalous effects can prove instrumental in the development of a field. Such anomalies are necessary but insufficient to convince a naturally skeptical scientific establishment that there is a there there.
Second, as an anomalous field quietly develops, it is important that supportive technologies and tangential, mainstream scientific advances, even if only recognized within the field, be identified and employed. In the case of the transistor, developments in materials characterization, single-crystal growth, and electron band theory all converged to elucidate the anomalous solid-state amplification effects. In the case of LENR, the emergence of quantum science, such as coherently enhanced chemical reactions and collectively accelerated nuclear state transitions, along with superior nanoscale materials development and characterization methods, should serve similar anomaly-clarifying functions.
When I presented ideas about LENR framed in the language of modern quantum dynamics models — developed over recent decades by Peter Hagelstein — at the European Physical Society Condensed Matter Meeting in Milan, Italy, they were perceived as a logical extension of principles already familiar to that community and met with curiosity and interest. Whereas, such presentations and discussions at a nuclear physics or engineering conference would likely be immediately controversial, met with a closed mind, and relegated as fringe.
Third, a well-resourced scientific coordination effort must synthesize these learnings, both tacit and conventional, and bring forth the highest quality scientific tools and methods to establish the anomalous effects beyond a reasonable doubt or to identify a set of the parameter space where anomalous data do not emerge. If the analogy holds, the ARPA-E program should play the role of this coordinating agent, akin to Bell Labs.
In the context of LENR, it is important to consider which types of experiments and empirical observables are likely to break the stalemate. Experiments with a track record of even moderate reproducibility and unambiguous nuclear observables are optimal (e.g., prompt particle radiation, transmutations with unnatural isotopic ratios, helium production, or significant excess heat with clear independent variable trigger stimuli).
An experiment that breaks the field open may look radically different from a potential future commercial LENR technology. That is because with increased advancement comes greater theoretical insights and technology development. And because, while proving the existence of a LENR phenomenon may optimize reactions for ionizing radiation production, which are comparatively easy to detect, the promise of LENR is, in part, the potential to decouple nuclear energy from ionizing radiation. The solid-state environment (e.g., metal matrix, doping, electromagnetic radiation stimulus, etc.) and condensed matter degree of freedom (e.g., phonons, plasmons, and magnons) could, in principle, engineer the underlying nuclear process and tune radiation production accordingly. This represents a radical departure from conventional principles of nuclear engineering.
Suppose such an experiment were to emerge and be published in a high-impact journal. In that case, I expect that while the orthodox narrative — that cold fusion is an illegitimate pathological science, would persist somewhat — it would matter little, as many unexpected mainstream research groups would promptly pursue LENR research, even if in its more contemporary cladding. It would be a race to a standard reference experiment with an accompanying theory.
Where does LENR fit into nuclear energy innovation?
How might LENR be expected to fit into the vast nuclear energy innovation ecosystem? The nuclear technology landscape can be thought of as a spectrum from low science risk but high engineering or deployment risk on one end, and high science risk but low engineering or deployment risk on the other. LENR is firmly on the latter end of the spectrum. But in a future scenario where LENR is shown to be a real scientific phenomenon, technology development could ensue quickly; much faster than its plasma fusion counterpart.
The articulated vision of LENR applications includes everything from cold fusion boilers to small-scale, factory-manufactured, solid-state LENR devices with immense power density and undetectable or benign (non-ionizing) radiation powering electronic devices. Imagine a phone or laptop that rarely or even never needs to be recharged during its usable lifetime.
If cold fusion is to break through, a well-coordinated research effort with premier scientific standards and capabilities, as well as tacit and explicit insights from the LENR field and its tangential scientific domains must come together in unison. With any luck, the ARPA-E LENR research program is exactly this effort. If that is so, we may be at the precipice of a nuclear science revolution and we don’t even know it yet.