Fusion Runs Hot and Cold
How the academy has gotten cold fusion wrong for over three decades
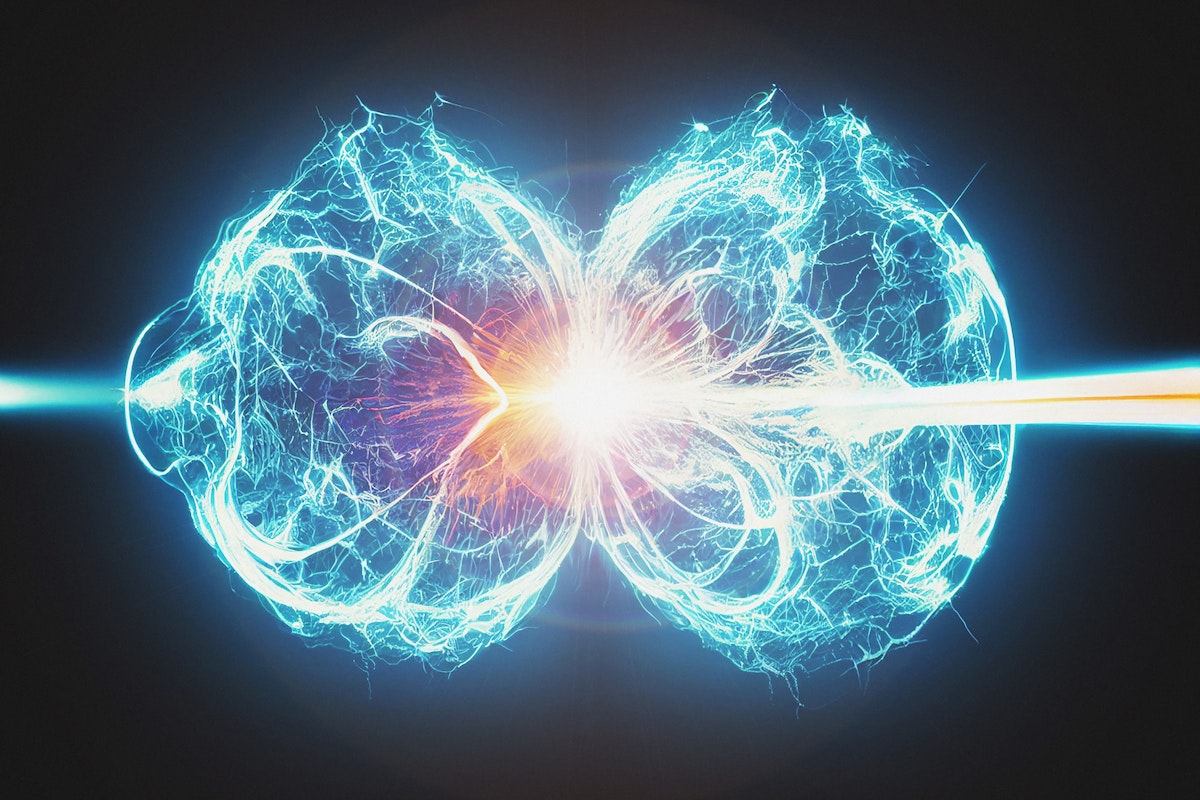
-
-
Share
-
Share via Twitter -
Share via Facebook -
Share via Email
-
Cold fusion is a topic sure to elicit at least a smirk from seasoned members of the academy. A third rail of physics often castigated as a “pathological science,” scientists and journalists alike regularly mock cold fusion in the most derisive and patronizing ways. Up until early 2022, I had internalized that understanding as well—that is, that cold fusion was a sort of pseudoscience promulgated without a technical basis. But that is categorically false.
My introduction to the field came via a Nature Perspective article, which summarized a research effort led by Google to revisit “the cold case of cold fusion.” The piece concluded without asserting proof for cold fusion, but it did highlight some interesting nuclear physics at low energies and opened my eyes to a field I had never taken seriously. That paper, discussions with some of its authors, and a deeper study of the field all pointed to one conclusion: to write off cold fusion simply because it does not readily comport with established frameworks of nuclear engineering is unscientific, deeply incurious, and stands in stark contrast with a large body of compelling empirical evidence.
Fast forward to March 2023, the U.S. Department of Energy’s (DOE) innovation arm, the Advanced Research Project Agency for Energy (ARPA-E), announced eight grant awardees to study low-energy nuclear reactions (LENR)—the DOE’s and the field’s preferred term for cold fusion. (I am a member of one of the awardee teams based out of MIT. We have made a version of our grant manuscript available to interested parties.) This development cannot be overstated: for the first time in 30 years, the DOE is funding cold fusion research. It is time for the broader scientific community and popular journalism to reject the palpable stigma that plagues this field and catch up with Washington.
And yet, from 30 years ago to the present day, one can hardly dare to engage in a serious conversation about LENR. Any uncertainty regarding the extent to which all standards of collegiality are thrown out of the window when it comes to the work of LENR scientists should be laid to rest by this piece for WIRED by Virginia Heffernan—a journalist and cultural critic—from earlier this year. Heffernan felt compelled, in an article about the exciting announcement at the National Ignition Facility of energy breakeven in “hot fusion,” to go on a scurrilous scourge for no apparent reason against cold fusion and its researchers likening them to an “aggrieved mini-cult” akin to “anti-vax doctors.” Virginia Heffernan has not, to date, responded to my critique and a standing open offer to explain my and my colleagues’ rigorous LENR ARPA-E research project.

The Cold Fusion Phenomenon—Then and Now
The story of cold fusion is widely misunderstood, as is the science underpinning the field. In March 1989, two highly accomplished electrochemists at the University of Utah, Martin Fleischmann and Stanley Pons, held a press conference claiming to have induced fusion reactions of deuterium nuclei (called deuterons, a heavy isotope of hydrogen with one added neutron) in a palladium foil. The palladium acted as the cathode in an electrolysis cell where heavy water (i.e. where the H in a H2O water molecule is replaced by deuterium) served as the electrolyte. When a current was applied to drive electrolysis, deuterons were absorbed into the palladium, forming a metal deuteride.
They ran several electrolysis experiments—inspired by long-since forgotten anomalous reports of cold-fusion-like experiments in the 1920s—for weeks at a time and reported several sharp, multi-day bursts in thermal power output from their cells well above the electrical power of the input current and well above the total potential energy stored in the chemical bonds of the used electrolyte. They measured this so-called excess heat using calorimetry—the process of quantifying the total amount of heat that escapes from a sample. Fleischmann and Pons concluded that the excess energy produced in their experiments was too great to be chemical in origin and instead suggested deuteron-deuteron (D-D) fusion as the source. Curiously, though, they did not observe the expected radiation. The two researchers admitted that an explanation for such a nuclear mechanism was elusive.
Their claims drew prompt and harsh criticism.
For one thing, Fleischmann and Pons announced their results by press conference rather than a peer-reviewed publication, which would not come until early 1990, a year after a preliminary note published in the spring of 1989.
Second, Steven Jones, a researcher at nearby Brigham Young University (BYU) was pursuing similar cold fusion experiments around the same time. The two teams had agreed to jointly publish their findings in Nature. The Fleischmann and Pons press conference happened eight days after they made their pact. Some observers have blamed their haste on pressure from the University of Utah’s administration, which seems plausible. Jones, though burned, submitted his results to Nature and continued a very convincing experimental campaign in the years that followed, reporting unambiguous neutron emissions above background from metal deuterides.
A third issue was Fleishmann and Pons’ reported gamma spectrum, which is summarized well in Steven Krivit’s book Fusion Fiasco. Conventionally, D-D fusion reactions emit neutrons, which, when stopped by protons in water used in a calorimetry system, emit characteristic gamma-rays (~2.2 MeV) that can be detected as secondary evidence for D-D fusion neutrons. The original submission of Fleischmann and Pons’ preliminary note contained a partial gamma spectrum with a peak at ~2.5 MeV, not seen in a control detector. After the energy discrepancy was pointed out to Fleischmann at a seminar, the manuscript was changed pre-publication to show the gamma peak at 2.2 MeV, which is a highly irregular adjustment for a journal to accept without explanation.
Scientists at MIT’s Plasma Science and Fusion Center published a blistering critique, noting that the peak was twice as narrow as expected at 2.2 MeV based on observed background peaks and that the spectrum lacked a compton edge, a feature of the physics of gamma-ray interaction with the crystal detector. They correctly concluded that the identified peak could not have been secondary evidence for the gamma-rays from D-D fusion neutrons. Fleischmann and Pons responded in Nature by publishing their full spectrum with the purported gamma peak above background back at the original ~2.5 MeV and acknowledged the previously erroneous peak at 2.2 MeV, which they explained as the result of a scaling and interpolation formula they used. They nevertheless affirm that the gamma peak is evidence for an unexplained nuclear reaction because the gamma peak does not appear in the background data and it is lost in the spectrum upon removal of their electrolysis cell. The MIT group responded in the same issue of Nature challenging the energy calibration and asserting that the gamma peak in question was likely at ~2.8 MeV, and wrote it and other high energy background peaks off as instrumental artifacts.
The lead author of the MIT team later said “they probably believed in what they were doing. But how they represented it was a clear violation of how science should be done.” The gamma-ray saga was sloppy. Fleishmann himself called it “rubbish” but he and Pons may have been on to something. The expectation that cold fusion would produce the same products as conventional fusion, and hence the critically important 2.2 MeV gamma rays, is off base.
The last problem with Fleishmann and Pons’ work is that, a lack of consistent reproducibility of excess heat combined with a lack of a theoretical explanation, effectively ended mainstream interest in cold fusion. By May 1989—not five weeks after the initial claims—the field was proclaimed dead by eight out of the nine main speakers at the influential May meeting of the American Physical Society (APS). Among the most impactful, Steven Koonin, then a professor of theoretical physics at Caltech—who is now better known for his dismissal of climate change impacts—mockingly proclaimed that “we’re suffering from the incompetence and perhaps delusion of Dr.s Pons and Fleischmann.” That fall, the DOE’s panel to evaluate cold fusion recommended against a federally funded cold fusion research program, but admitted that some evidence (e.g. neutron and tritium production) was not so easily dismissed.
Even though the majority of the scientific community had written off cold fusion after only a few months, a growing body of scientific evidence of objectively nuclear reaction products (e.g. neutrons, gamma rays, and tritium) would mount in the ensuing months and years. Even some skeptics found the data impossible to ignore, and critical scientists occasionally even acknowledged that “there were phenomena described to us [the first DOE panel] where you could not offer alternative… explanations.” This begs the question, “what if cold fusion is real?”
In 2004, the DOE agreed to take another look at cold fusion given the evidence that had accumulated over the previous decade. These results were curated in a report prepared by leading LENR scientists. The reviewer response was mixed, and the DOE maintained that it would, in principle, be willing to fund high-quality grant proposals. But up until this March, not one LENR project had actually been funded by the department.
All this, despite outspoken support for LENR research by the likes of Edward Teller and Nobel Prize winning physicists Julian Schwinger and Brian Josephson. In fact, Schwinger’s conviction was so strong that when the APS refused to publish his papers on cold fusion in their journals without giving them proper consideration, he revoked his prestigious APS fellowship, commenting “the pressure for conformity is enormous… the replacement of impartial reviewing by censorship will be the death of science.”
Fleischmann and Pons have wrongly become the poster children for fraudulent or “pathological science,” an assertion first leveled by CERN physicist, Douglass Morrison. Yet one need not rely solely on the Fleischmann and Pons results to be convinced of a genuine LENR effect. Indeed, the most compelling evidence for LENR has essentially nothing to do with excess heat at all.
What are the Common Critiques of Cold Fusion?
The obvious counterargument goes like this: how could it be that some small community of LENR researchers is right about this niche field and the entire physics community has meanwhile been dead wrong for over 30 years? That is certainly a valid question deserving detailed exploration.
In general, such critiques of cold fusion fit into two groups. First, that claims of cold fusion are tautologically inconsistent with prevailing nuclear theory. Second, that experiments are anomalous and hard to reproduce.
Two particular theoretical gripes face cold fusion. Fusion reactions of positively charged hydrogen nuclei must overcome the coulomb barrier—the electrostatic repulsion of like charges—requiring particle energies of at least ~2 kiloelectron volts (KeV). In conventional “hot fusion” this is accomplished by increasing the temperature of hydrogen plasma to tens or even hundreds of millions of degrees to get hydrogen nuclei close enough for the strong nuclear force to overpower coulomb repulsion. Thus, how can the coulomb barrier be overcome to achieve observable fusion rates at ambient temperatures?
Next, plasma fusion reactions have been studied for decades both in the lab and in stars. Put simply, we know what particles to expect, at what energies, and in what proportions from a wide variety of fusion reactions. Conventionally, the D-D fusion reaction has three pathways with relative likelihoods—known as branching ratios—as shown in Figure 1. Cold fusion experiments do show a variety of radiation emission characteristics but never all of the expected products, let alone in the anticipated proportions and absolute amounts. So if cold fusion was occurring in these experiments, where are the expected nuclear products?
Indeed, a Caltech physicist wasquoted as reacting to the Fleischmann and Pons experiment by saying “It’s bullshit… if it were true, they’d both be dead.” Based on the heat production claimed, the neutron fluxes from the D + D → He-3 + n branch should have been fatal. At first glance, it’s a reasonable concern and the absence of expected fusion reaction products seems quite damning—but upon further examination, this objection is deeply ignorant.
Diagram of D-D fusion reaction branching ratios and corresponding characteristics
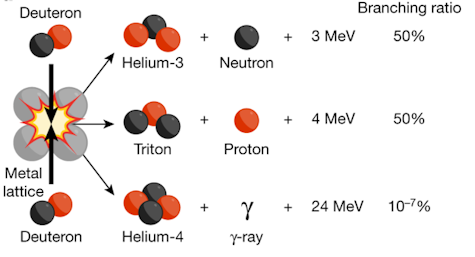
From first principles, nothing necessitates these particular reaction pathways and branching ratios. The fundamental physics principles that cold fusion must satisfy are the conservation of energy, momentum, and various particle and quantum number characteristics. Consider how dropping a marble in an empty glass will make a loud thud, whereas if the glass is filled with water, the marble will dissipate its energy in the liquid, resulting in a far less audible impact. Similarly, a metal lattice can, in principle, absorb the nuclear binding energy from a fusion reaction via lattice vibrations, for example, as opposed to that energy manifesting via high energy particles (i.e. the loud thud) as is the case for plasma-based fusion.
To be sure, fusion reactions in metal targets at low energies do produce conventional products, but it is not inconceivable that under certain conditions (e.g. quantum coherence) fusion reactions could take place without yielding expected plasma fusion products. This is reminiscent of the Mössbauer effect. Normally when a nucleus emits a gamma-ray, it fiercely recoils to conserve momentum. However, in a solid lattice, the recoil is absorbed by the entire crystal resulting in negligible energy loss of the gamma-ray and a sort of periodic resonant emission and absorption process.
The two canonical articles written in 1989 that ostensibly rule out cold fusion—a Nature article by Koonin and Nauenberg and a Physical Review Letter by Leggett and Baym—both ignore quantum coherent effects. Well not quite. Leggett and Baym actually explicitly state that they ignore an “exotic… coherence between fusion processes involving different deuteron pairs.” But how ‘exotic’ would such effects actually be? In their rush to quash the field before it even started, neither article bothered referencing a Physics Review Letter published 24 years prior to any cold fusion drama, which, under conditions similar to those in cold fusion experiments, suggests exactly such coherence effects.
The plasma environment is wildly different from a metal lattice. And there are legitimate reasons to believe that nuclear reactions can operate very differently in the latter.
That’s all well and good, but one might then ask: why aren’t these experiments widely and easily reproduced? There are two answers. Defining what qualifies as a replication attempt is crucial when working in the solid state environment and especially if coherence effects are at play. For example, a later experiment by Jones at BYU, in collaboration with Los Alamos National Laboratory (LANL), reported anomalous neutron bursts from titanium samples in deuterium gas significantly (as much as two orders of magnitude) above background radiation, which was absent in control data. When a team from Yale and Brookhaven National Laboratory failed to reproduce the experiment, doubt was cast on the cold hard evidence for a nuclear process.
Jones responded by pointing out that the limited run time of the Yale and Brookhaven Laboratory experiments was statistically unlikely to yield the sought-after results. But what was left unmentioned, and is far more damning, is that the Yale-Brookhaven team, by their own admission, didn’t remove the titanium oxide layer from all but one of their samples, functionally prohibiting any significant absorption of deuterium gas. Such a critical omission prevents this study from truly qualifying as a replication experiment.
And indeed anomalous neutron and gamma-ray emissions were subsequently reported by the BYU-LANL team and a BYU-Italian collaboration and by groups in Russia, New York, and two groups in Italy. The BYU-LANL team would later withdraw their claims due to a later failed replication experiment and assign the anomalous effects to an erroneous detector. Yet a malfunctioning detector then does not explain why the clearly positive results do not appear in any of the accompanying control experiments or background data. Almost a decade later, Jones published another replication study, which unequivocally showed that observed neutron data were genuine and emanated from the metal deuteride sample. But by then, cold fusion had been excoriated as junk science and very few researchers likely read the new paper.
Stepping back from the theoretical disputes over cold fusion and conjectured theoretical explanations, experimental observations must remain the principal metric in assessing the validity of LENR. Physical theories are only correct in so far as they agree with nature. No LENR theory is recognized as fully explanatory, but some compelling theoretical frameworks (link not regularly updated nor complete) directly refute many common theoretical objections. And while reproducibility of experiments is crucial for scientific advancement, examples abound of scientific phenomena such as semiconductor amplifiers and transistors that, for reasons that make perfect sense in hindsight, posed reproducibility challenges for decades.
What are the Central Claims of Cold Fusion?
Researchers often misidentify what the central claims of cold fusion–more precisely LENR—actually are in the first place. The vast majority of scientists are either unaware of the field at all or understand the central claim to be solely limited to the excess heat generated in Fleischmann and Pons-like electrochemistry experiments. This perception is simply incorrect.
The commonly known result, excess heat, is ambiguous in its nuclear origin. Meanwhile, lesser known but well-documented phenomena such as high-energy particle emission with resonance peaks and nuclear transmutation products with unnatural isotopic ratios are far more compelling and clearly indicate nuclear reactions.
In practice, the central claims of the LENR experimental literature are the following:
Excess heat (i.e. excess thermal power and energy)
High energy (≥KeV) gamma-ray, neutron, and/or charged particle emission*
New low-atomic number element production that appear to be fission products, which are often correlated to morphological features (e.g. craters) on metal hydride surfaces
Particle capture transmutations by metal hydride dopants
Unnatural isotopic ratios in new element transmutations and host metal nuclei
Whereas conventional nuclear reaction products are consistent and predictable, LENR experiments report a confusingly disparate set of results. Making sense of the field therefore represents a genuine challenge. For example, many studies report particle emission from metal hydride samples in out-of-equilibrium conditions, but these particles vary widely in kind (neutrons, alpha particles, and more) and in energy (KeV to MeV).
Ambiguous nuclear origins aside, excess heat tells an observer essentially nothing about the nature of the purported nuclear reaction, providing limited scientific insights. On the other hand, new element detection, isotopic shifts, tritium production, and particle emission can reveal distinctive patterns and better elucidate the nature of potential nuclear reaction mechanisms. A large collection of such well-controlled experimental results have been best summarized in two books by Edmund Storms, a retired long-time LANL research scientist.
It is therefore curious that claims of cold fusion have been framed so derisively in the general scientific community (see Douglas R.O. Morrison’s and Robert F. Heeter’s comments), with the best empirical evidence having gone largely unread or misconstrued. Claims as big as cold fusion often encounter sharp critique, to be sure. A favorite quote of critics asserts: “extraordinary claims require extraordinary evidence.” In popular usage, many often fail to credit this quote to Marcello Truzzi who, as Brian Josephson pointed out in his Nobel Laureate lecture, later reflected that the sentiment was “a non sequitur, meaningless and question-begging.”
What is the path forward on fusion?
A nuclear energy advocate recently asked me “what’s your personal optimistic take on… LENR.” My simple answer was that LENR could be to contemporary nuclear technology as the transistor is to vacuum tubes. But the full answer is more complicated. It may be the case that while real, cold fusion will never be a viable energy technology because LENR effects may occur only in isolated regions of metal hydride surface layers. But if proven and extended to a larger volume of the metal, the applications could be tantalizing.
Researchers have much work to do. LENR remains shunned and ignored by mainstream institutions of science, and while the field is experiencing an exciting resurgence in interest, it still suffers from what the philosophy of science professor Huw Price calls a “reputation trap” that dissuades new entrants into the field.
If cold fusion is eventually proven to be real, I anticipate that the broader scientific community’s response will almost certainly be to perform an about-face, arguing that the scientific establishment had never totally ruled out cold fusion and that in the end, science prevailed, rather than to seriously reckon with why science had failed for so long. In the meantime, LENR or cold fusion is, unfortunately, likely to remain a verboten topic until the field’s principles are proven in an irrefutable reference experiment published in a prominent journal. Until then, we will be in our labs working as best we can.
*This line has been edited to replace "(>KeV)" with "(≥KeV)"
Originally published May 18, 2023, updated to fix a typo April 22, 2024.