What the 100% Renewables Literature Gets Wrong
Claims that Asia and Africa can easily achieve a clean energy transition at low cost using renewables and storage alone are bunk
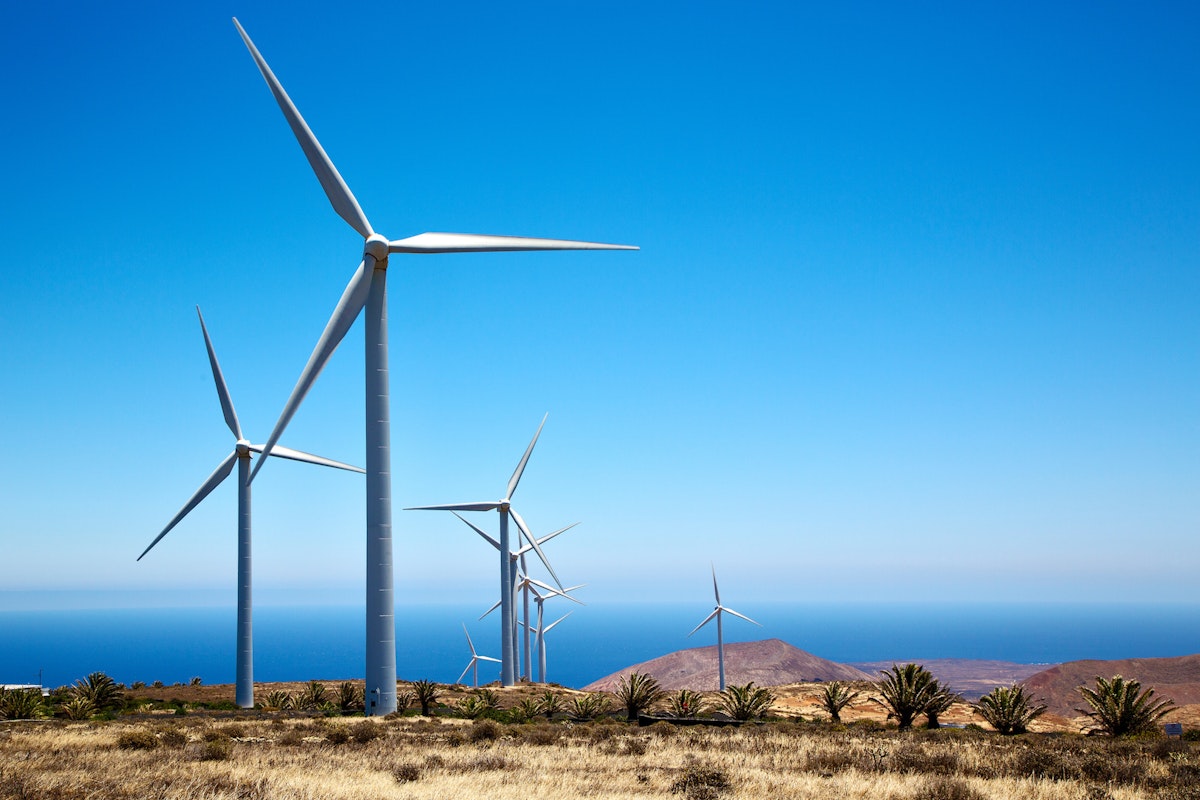
-
-
Share
-
Share via Twitter -
Share via Facebook -
Share via Email
-
This article text, Table 1, and Figures 2 and 3 have been revised in order to correct a factual error in which the author mistook 2030 cost input assumptions in several papers as 2020 cost input assumptions. Revisions have been kept to the bare minimum needed to correct this misinterpretation and specific statements that invoked these values as evidence. A full document listing all tracked changes, with original and revised table, figures, and text shown side-by-side, can be found here.
Executive Summary
“A global transition to 100% renewable electricity is feasible at every hour throughout the year and is more cost-effective than the existing system… Energy transition is no longer a question of technical feasibility or economic viability, but of political will.”
So goes a mantra common to much of the environmental NGO and advocacy community, particularly in Europe and the Anglosphere. It’s half true: recent years have seen remarkable cost improvements for wind and solar energy and battery storage, and these technologies will undoubtedly supply large shares of future electricity generation. Policymakers, especially in Europe and North America, now possess many potent clean energy options to deploy in support of climate efforts.
But often, advocates take recent cost improvements for wind, solar, and batteries to ideological extremes, seeking to frame renewables and storage as the only permissible technology options for future energy systems. Furthermore, they frequently extrapolate these preferences to distant corners of the world.
Some argue renewables and storage are already sufficiently cheap to enable poor countries in Sub-Saharan Africa to achieve their economic development goals while leapfrogging any further use of fossil fuels. Others go so far as to assert that countries like South Korea or Japan ought to phase out their substantial domestic clean nuclear generation capacity and focus future energy policies exclusively on new wind, solar, and storage technologies. To support these claims, advocates often cite a group of academic energy system modeling papers that appear to tell them exactly what they want to hear: that humans across the globe can begin affordably transitioning to electricity systems that use renewables and storage alone.
For countries like Norway, Iceland, or New Zealand, blessed with small, wealthy populations and an abundance of hydropower or geothermal energy, transitioning to fully renewable electricity systems may be both feasible and cost-optimal. But many countries—especially densely populated and/or developing nations in Africa and Asia—are not so fortunate. Based on first principles alone, the claim that most of these countries can easily achieve a clean energy transition at low cost using renewables and storage alone deserves rigorous scrutiny.
Figure 1: World map of cited 100% RE system studies
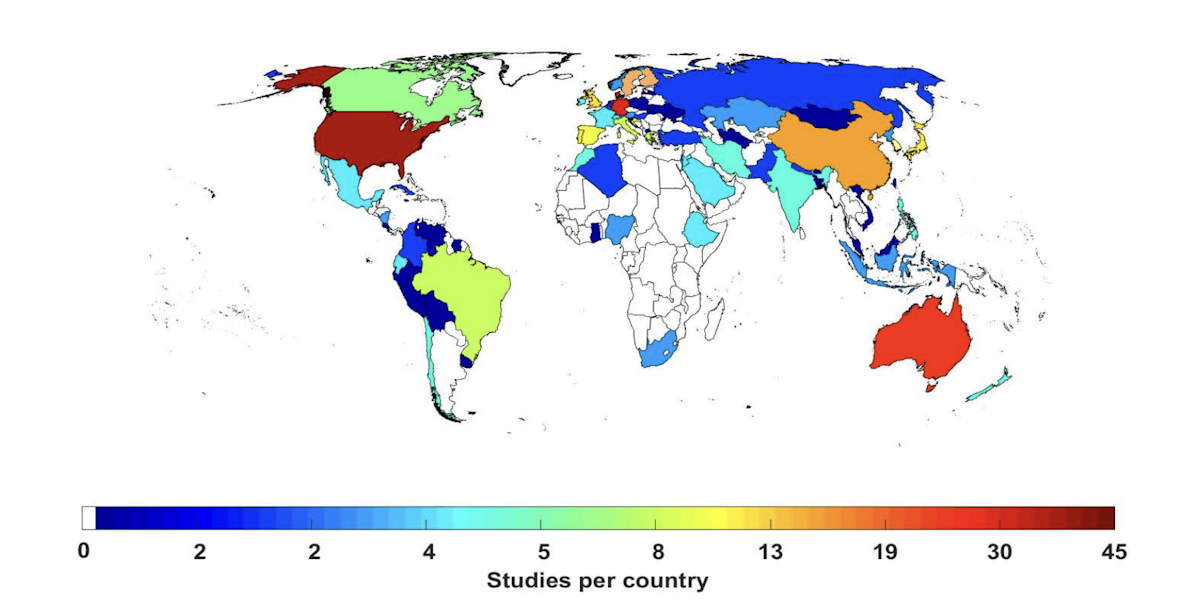
In July 2022, a group of prominent 100% renewable energy (RE) scholars published an overview of the 100% RE literature in the journal IEEE Access titled “On the History and Future of 100% Renewable Energy Systems Research.” “The main conclusion of most of these studies is that 100% renewables is feasible worldwide at low cost,” the abstract declared. In November 2022, two of those researchers (Khalili and Breyer) published their bibliometric analysis of over 600 academic articles that they claim support the conclusion that 100% RE systems are not only feasible but cost-efficient—for countless countries and regions across the globe (Figure 1).
I have reviewed dozens of the articles cited by Khalili and Breyer, focusing specifically on the 86 allegedly demonstrating region, country-level, and sub-country-level 100% RE systems in Asia (61 papers) and Africa (25 papers). On closer examination, I found that this body of literature exhibits pervasive, serious methodological flaws. These weaknesses cast doubt on dozens of regional and country-level studies often cited in the policy discourse to claim that countries in Asia and Africa can transition easily and affordably to 100% renewable energy systems.
Key findings:
- Much of the literature on 100% renewable system studies for Asia and Africa has been published by a single research team, using a single simple energy system model. A full 38 of the 86 papers (44% of the total) cited in Khalili and Breyer’s bibliometric analysis are reports of studies led by German-Finnish researcher Christian Breyer and utilize the Lappeenranta-Lahti University of Technology (LUT) Energy System Transition Model with highly problematic energy system cost assumptions.
- The Breyer group’s employment of the LUT Energy System Transition Model vastly underestimates the costs of 100% RE electricity systems across Asia and Africa for the following reasons:
- The research team often assumes aggressively cheap renewable technology costs, even for present-day projects.
- These studies assume the cost of capital is identical (7% for utility projects) in all markets and that energy project capital costs are extremely cheap even for emerging economies.
- The LUT model only deploys resources to match anticipated electricity demand, without accounting for operating reserve margins.
- The LUT model assumes a simple nodal grid, omitting many crucial transmission, distribution, pipeline, storage, and system costs and constraints, including infrastructure for ancillary services.
- The LUT model assumes perfect meteorological forecasting and omniscient dispatch and grid operation, further underestimating overbuild of generation, storage, and transmission resources.
- The LUT model appears to rely heavily on many technologies that are of questionable climate benefit or that have not yet scaled commercially, including biomass energy, thermal energy storage, and compressed air energy storage, and notably on the assumption that direct air CO2 capture and hydrogen electrolyzers can produce affordable net-zero “synthetic natural gas” to power backup gas generators and fill seasonal storage
- The other 48 papers for the Asia/Africa region exhibit numerous problems and limitations:
- 15% contain minimal or no original calculations at all (13 papers, including qualitative discussion and review papers, with one extremely poor-quality “machine learning” paper, are cited three times in the context of different countries).
- Another 15% either contradict the assertion that 100% RE systems are feasible and cost-effective, do not model full power sector decarbonization, use only simple spreadsheet models, or are erroneously cited.
- Most of the remaining studies, like the papers published by Christian Breyer’s group, also use simplistic energy system models that cannot serve as a basis for national power sector planning.
- Finally, virtually none of the 86 cited papers on 100% renewable system studies for the Asia and Africa regions actually conducted separate system-level cost modeling for alternative scenarios, such as more energy-intensive scenarios or policy approaches that allow construction of nuclear power plants, power plants with carbon capture technology, or backup gas generators. As such, the claim in the 100% RE literature that 100% RE systems are cost-optimal relative to systems that include nuclear, carbon capture, or backup gas remains largely unsubstantiated.
In summary, stakeholders in Asia and Africa in particular should recognize such limited research papers for what they are: simplistic studies with implausibly optimistic assumptions. Sufficiently simple models using sufficiently unrealistic inputs can generate amazing outputs. But they will have next to no utility in real-world decision-making and are hence dangerously misleading for informing policy. Asian and African policymakers and environmental advocates would thus be prudent to more critically scrutinize rosy research articles in the 100% RE genre.
Asian and African governments and researchers should instead commission and perform more rigorous, objective energy system capacity expansion modeling studies that can more accurately and impartially identify cost-efficient paths forward for domestic power sector decarbonization.
1. The LUT Model's original sins
1.1 Half of the 100% renewable energy literature for Asia and Africa stands on a single model
The body of 100% renewable energy (RE) systems literature for Asia and Africa is surprisingly shallow. A full 38 of the 86 Asia- or Africa-related papers (44% of the total) cited in Khalili and Breyer’s bibliometric analysis are reports of studies led, co-authored, or supervised by German-Finnish researcher Christian Breyer at the Lappeenranta-Lahti University of Technology (LUT) in Finland and utilize the LUT Energy System Transition Model with only small adjustments for regional differences.
LUT model studies from Breyer’s research team account for 10 of the 25 Africa region studies and 28 of the 61 Asia region studies. This makes a concerning proportion of the Asia and Africa regional 100% RE systems literature dependent on work from a single research group, using a single simple, generalized model.
The authors of the historical review article surveying over 600 global 100% RE studies themselves discuss how much of Asia and Africa remains highly understudied regarding this energy sector. Given that energy system models all have their own strengths and weaknesses and are highly sensitive to input assumptions, this imbalanced literature at the very least suggests that clean energy systems research in Asia and Africa is in dire need of additional study, particularly involving corroboration across different scenarios and cross-validation using alternative models and methods.
Click for interactive table: ASSESSED 100% RENEWABLE LITERATURE FOR ASIA AND AFRICA
The dominance of the LUT model in the Asia and Africa 100% RE literature makes critical evaluation of this one team’s work all the more important. As subsequent sections will illustrate, closer inspection of these studies reveals significant shortcomings in the LUT team’s approach and highlights the acute need for additional and potentially competing research examining these regions.
1.2 The LUT model has anomalously low renewable technology cost assumptions
A major flaw of many LUT model papers involves the use and re-use of renewable energy project cost assumptions that are conspicuously lower than more commonly used cost inputs used elsewhere in the energy system modeling literature. For instance, some of the cited LUT model studies assume that the capital costs of utility-scale solar systems in 2020—in developing countries and high-cost East Asian countries like South Korea or Japan—are 68%-82% ($707 to $863/kW(DC)) of what the National Renewable Energy Laboratory’s 2023 Annual Technology Baseline (NREL ATB) datasets assume that large solar farms cost in 2021 ($1047/kW(DC)) in the United States.
The same pattern appears in LUT model wind project cost assumptions ($1555/kW), which are within 6% of the model input cost assumptions the 2023 NREL ATB recommends ($1462/kW) for onshore wind projects in the United States. Even from a global perspective, these costs fall on the lower-middle end of government estimates, modeling assumptions, and real project costs produced by researchers and industry today (Figure 2 and Figure 3). Such cost input values are not appropriate for modeling clean energy deployment in high-cost Asian markets or in developing countries.
Figure 2: Total installed costs by country for utility-scale projects, 2020
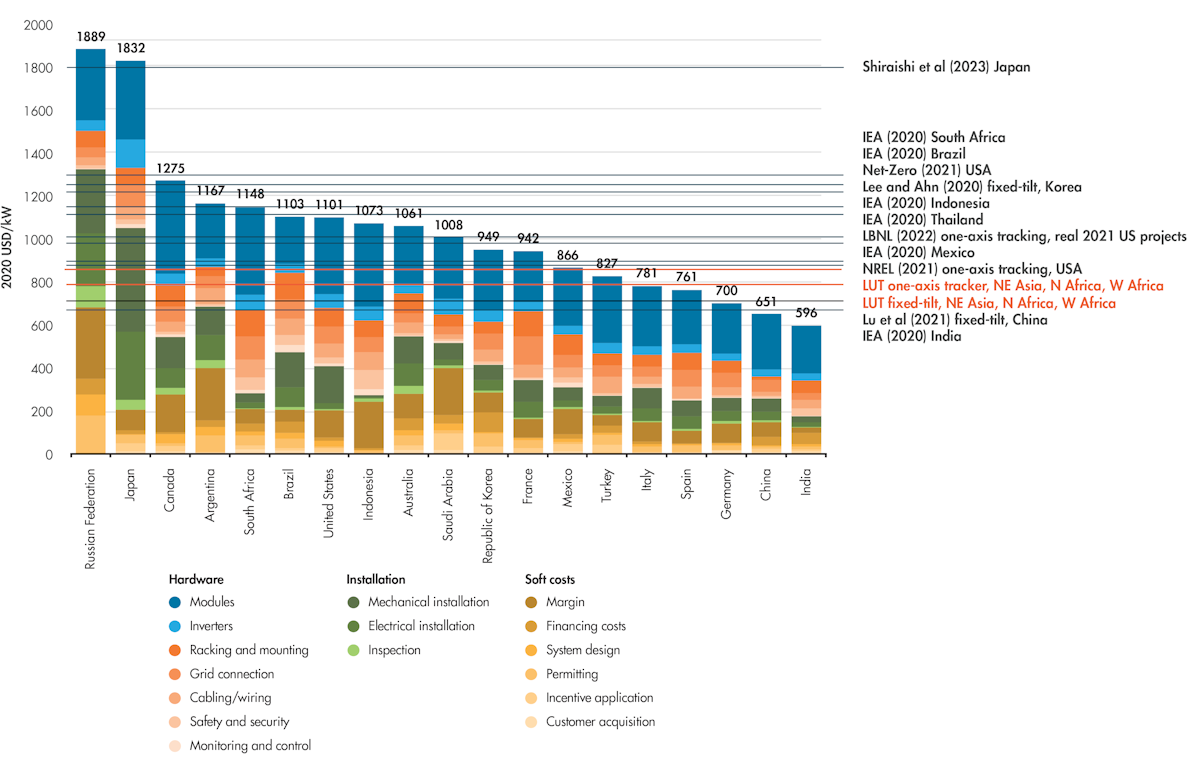
Figure 3: Total installed costs by country for utility-scale projects, 2020
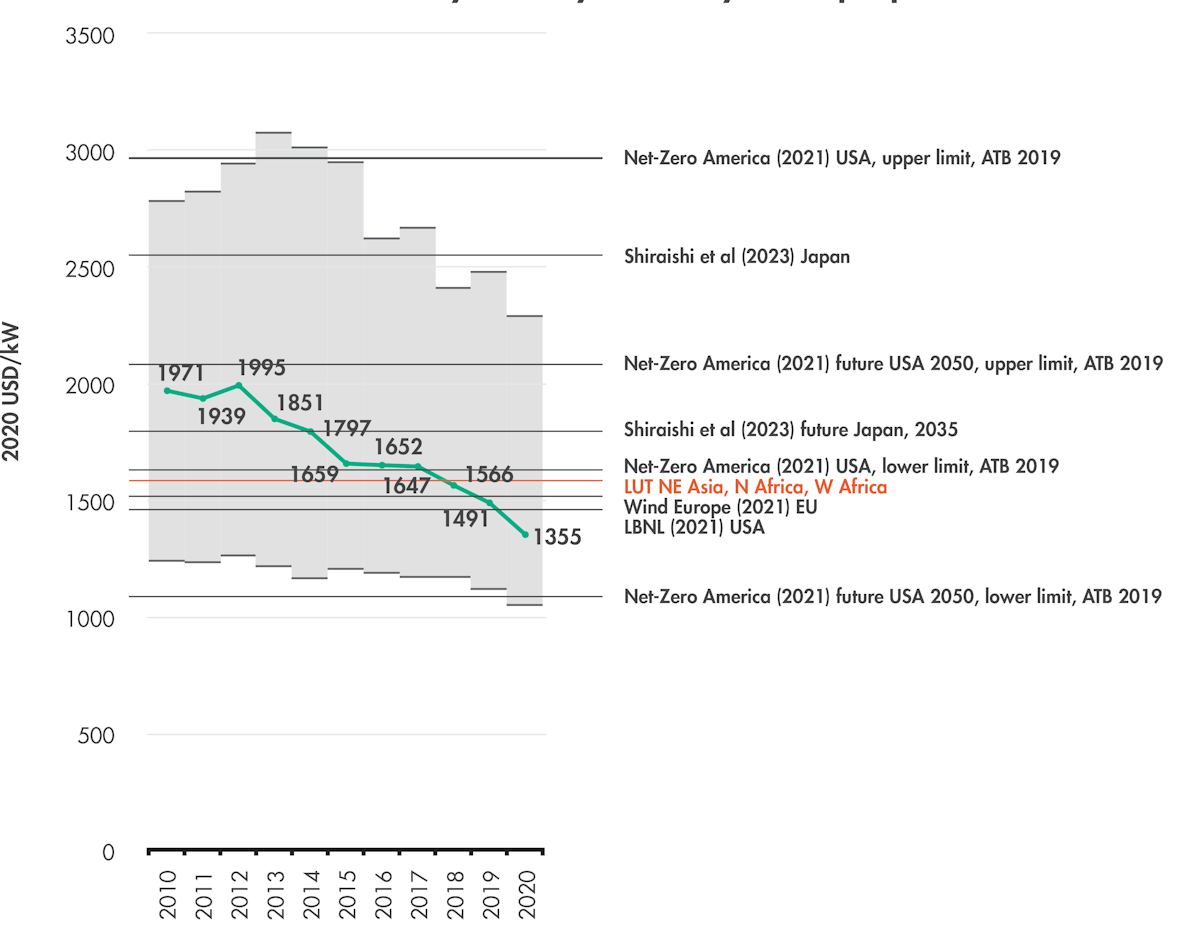
Certainly, countries like Japan or South Korea, where renewable projects currently confront higher costs, can see dramatic cost improvements as deployment accelerates. But the LUT team’s assumption that such countries can install new clean energy capacity today at the same global average costs assumed for wind or solar projects is clearly aggressive.
Meanwhile, despite common knowledge that energy infrastructure costs are considerably higher in many low- and middle-income countries, LUT model papers on Sub-Saharan Africa assume that natural gas power plants cost the same there as they do in China or South Korea and that rooftop solar, utility solar, and lithium-ion battery storage projects in Ethiopia and Turkmenistan
actually cost less than they do in Asia (Table 1). To be fair, some of the cited LUT papers do conversely assume higher costs for biomass and synthetic methane infrastructure in some contexts.
These papers similarly apply identical costs of capital to most of their studies. From Kazakhstan to West and Sub-Saharan Africa to Bangladesh to Iran to Northeast Asia, the LUT team assumes a uniform 7% weighted average cost of capital for utility-scale projects. In practice, developing countries often face significantly higher costs of capital.
Table 1: Table 1: Assumed energy project capital expenditure (CAPEX) costs in selected LUT modeling studies
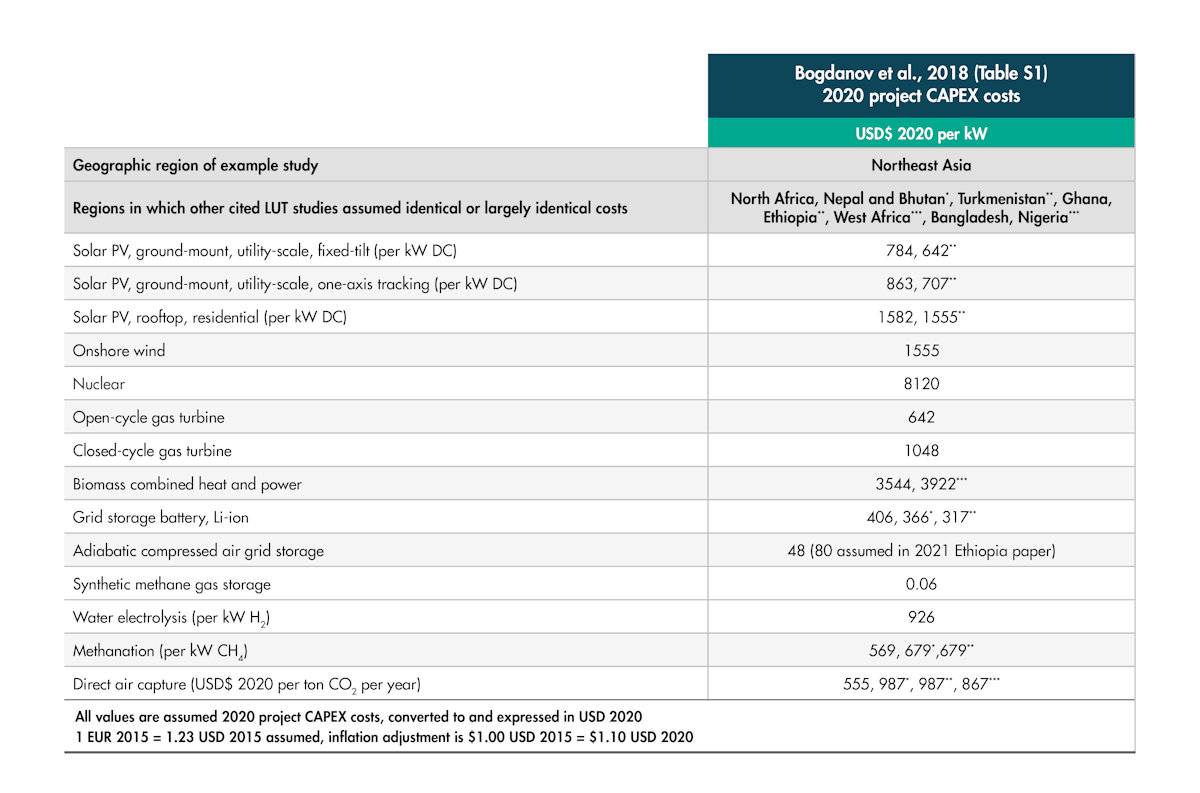
While solar, wind, and batteries provide much of the projected future energy mix in LUT model studies, these researchers also assume cheap costs for other supporting technologies. Such low input cost values likely make it far easier for the model to arrive at a low-cost 100% RE solution. Adiabatic compressed air energy storage costs far less than lithium-ion battery storage, but the researchers make no evident effort to assess the true availability of underground caverns suitable for compressed air systems in each study’s context.
Most notably of all, 100% RE systems in LUT model papers often rely on a small but important backbone of gas-fired power plants, which allegedly consume "RE-based synthetic natural gas (SNG) with zero net GHG emissions, as the CO2 is provided by direct air capture units." RE-based synthetic natural gas relies on electrolytic production of low-carbon hydrogen combined with electricity-intensive direct air capture of atmospheric CO2, with a subsequent chemical process combining these feedstocks to produce synthetic methane that power plants can burn like conventional fossil gas. No such technology exists at commercial scale today, and the economic viability of SNG depends critically on the costs of hydrogen electrolysis, direct air capture, and methanation, as well as infrastructure like belowground gas storage facilities.
The LUT researchers assume some of these costs to be quite low in some studies. Papers on Northeast Asia, Ghana, and Bangladesh model direct air CO2 capture capital costs starting at around $555/ton capacity in 2020 (2020 dollars) (compared to rates of $600-$1000/ton offered by direct air capture pilot firms today). Other studies on the Himalayas, Ethiopia, Nigeria, and West Africa do assume higher costs of $867-$987/ton, although this is still effectively on par with the global state-of-the-art in wealthy countries. Their electrolyzer costs in Sub-Saharan Africa are $926/kW in 2020, when much of the literature assumes current global costs of around $760-$1200/kW. Such low cost inputs raise the question of how sensitive LUT model total system cost results would be to higher starting and future cost assumptions.
1.3 The LUT model relies on speculative technologies and biomass energy to balance variability
These modeling assumptions for direct air capture and synthetic natural gas in turn raise questions regarding the LUT team’s decision to include highly speculative or dubiously sustainable technologies in 100% RE systems. No commercial power plants operate using 100% renewable synthetic natural gas today. Despite ruling out conventional and advanced nuclear power on the basis of cost and technological readiness, the Breyer team relies heavily on the viability of yet-hypothetical large-scale direct air capture and methane production from renewable hydrogen.
One LUT team modeling paper examining East Asia illustrates this contradictory frame of thinking in a pair of back-to-back sentences in the abstract: “Australia could become a liquefaction hub for exporting RE-SNG to Asia and a 100% renewable energy system could be a reality in East Asia with the cost assumptions used. This may also be more cost-competitive than nuclear and fossil fuel carbon capture and storage alternatives.” Not only is renewables-to-gas technology spared the skepticism leveled at nuclear and carbon capture technologies, but the authors presume the economics are favorable even after factoring in the logistics of transoceanic shipping, which the study itself does not quantify. Khalili and Breyer’s bibliometric review compiling hundreds of 100% RE papers highlights this contrast, lumping nuclear power together with fossil fuels in describing articles on the “transition from a fossil-nuclear energy system to a 100% renewables-based system” only to praise power-to-gas systems as a “major new concept” in 100% RE systems analysis in the very next sentence.
Essentially, this focus on renewable synthetic gas permits the LUT model to build combined and open cycle gas power plant capacity to balance the energy system, using generous input assumptions to argue that these plants will operate economically using renewable methane only. Electrolyzers, direct air capture facilities, and methanation plants appear to operate economically in the LUT model despite relatively low usage rates for such capital-intensive infrastructure (Figure 4).
Figure 4: Example of LUT results illustrating a modeled 100% RE power system for Kazakhstan
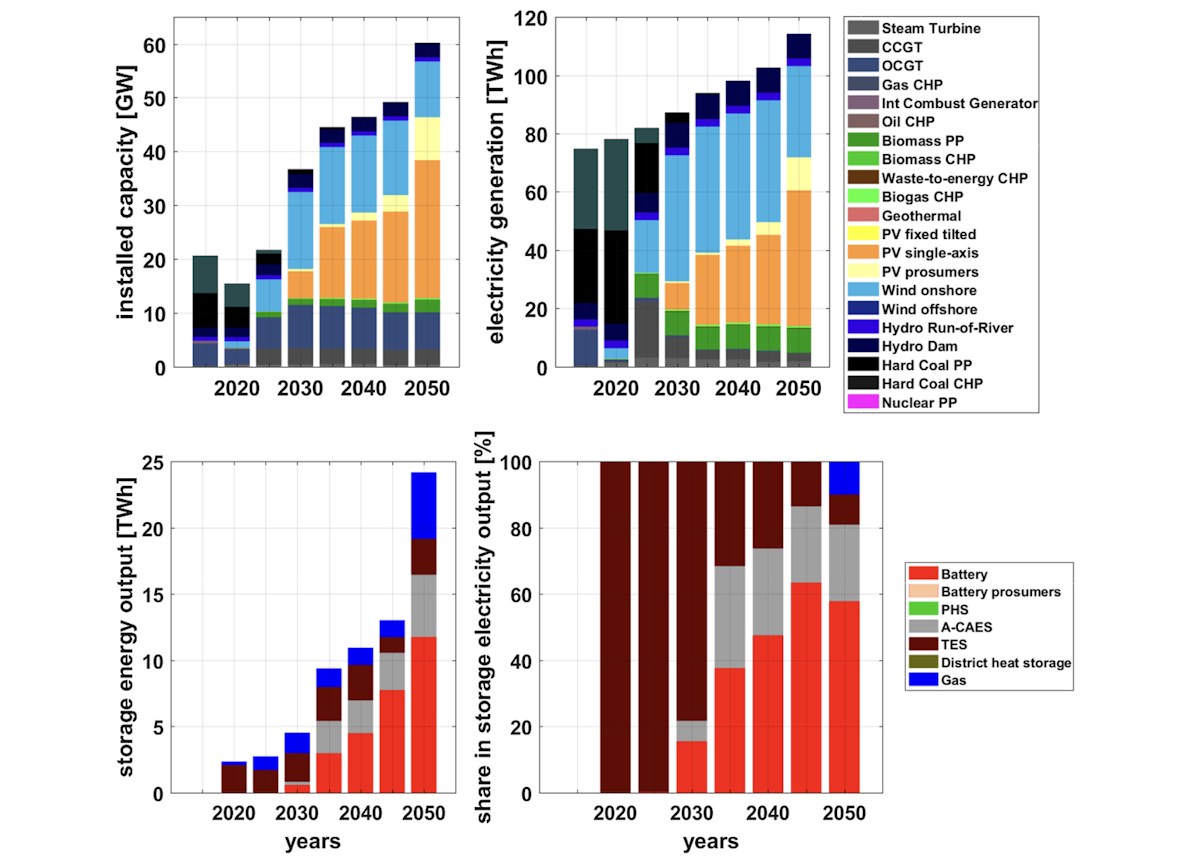
Some studies, such as those described in 100% RE papers for Kazakhstan and India, also leverage sizeable quantities of biomass energy, which supplies 5%-10% of electricity in 2050 in some models. Extensive research and reporting have highlighted serious concerns regarding the environmental and climate impact of burning biomass or waste to generate electricity, including air pollution, the use of suspect and unsustainable fuel feedstocks, and the higher-than-desired carbon footprint of biomass energy.
Given the importance of flexible assets for balancing daily and seasonal fluctuations in variable renewable generation, both modeled system feasibility and cost depend heavily on these synthetic gas, storage, and biomass assumptions. Basic sensitivity testing of LUT model 100% RE system claims could thus start by evaluating the ability of the model to produce a feasible, cost-efficient 100% RE solution at all, after raising cost assumptions for synthetic natural gas or excluding SNG, biomass, or other speculative technologies.
1.4 A simple model approach does not capture routine operational challenges
The powerful influence of very low input costs aside, the LUT model also likely yields low system costs due to its omission of basic operational factors that would necessitate deployment of additional generation resources in practice. For instance, the LUT team’s methodology only matches available modeled electricity supply to projected demand, without considering reserve margin requirements that would entail additional infrastructure investment. Other clean electricity modeling studies typically incorporate such reserve requirements—or at least maintain firm generating capacity to bolster grid reliability.
Reserves form a critical component of real-world grid operations for several reasons. An operational reserve margin accounts for system failures and the possibility that some generation resources cannot produce as planned. In particular, increasing penetration of weather-dependent renewables like solar and wind increases the sensitivity of generation to even improving, shrinking errors in forecasting. This factor is especially important to consider for smaller countries or regions with few or no interconnections, for which solar and wind conditions may be uniformly lower than anticipated across the entire system. Planning reserves, in contrast, compensate for uncertainty in system-wide projected demand.
Certainly, the Breyer team may deem factors like forecast uncertainty and reserve margins beyond the scope of their analysis; but if they do, they must appropriately qualify their claims of feasible and cost-effective 100% RE systems and acknowledge that their methodology omits many real-world considerations that might substantially increase the total cost of their proposed 100% RE system in practice.
1.5 A simplistic modeled network underestimates system costs
Claims that 100% RE systems are possible worldwide at low cost also typically assume highly simplified electricity networks. Despite the fact that 100% RE systems necessarily rely upon more spatially distributed generation resources, greatly increasing transmission network complexity, the current literature generally considers only simple nodal systems at best, with all generation and consumption concentrated at a single point in each region. Some studies assume even simpler systems: unconstrained and lossless transmission, or even just a one-region representation.
The LUT model adopts a simple nodal structure that considers transmission constraints and losses only along the single pathways linking adjacent nodes. Although the Breyer team has readily applied this structure to a wide array of regions and countries, such a model formulation is fundamentally limited in its ability to realistically represent the grid in the region of study. In one series of similar papers on a 100% RE “Super Grid” for Northeast Asia, the team represents South Korea as a single node, Japan as two nodes, and East China—a region whose population of 380 million well exceeds that of the United States—as a single node (Figure 5). One might also note the researchers’ politically optimistic interest in the feasibility of North Korean transmission corridors linking China, South Korea, and Japan.
While common in country-scale simple modeling studies, such low-resolution spatial structures clearly underestimate the true system costs associated with interconnecting wind and solar projects within entire provinces and countries, while greatly simplifying challenges associated with land availability and renewable project siting. Crude network structure also omits important supporting operational infrastructure like grid-forming inverters and synchronous condensers. Despite CO2, hydrogen, and natural gas pipelines being key to the LUT model’s envisioned “renewable” synthetic natural gas plants, it does not model costs or constraints associated with such infrastructure.
Figure 5: Simple nodal spatial configuration used by LUT model studies
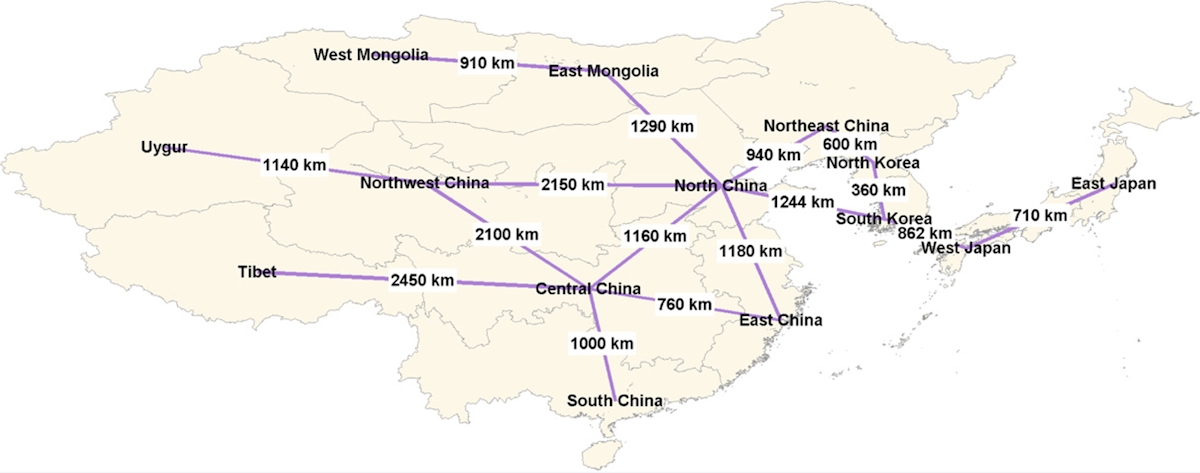
These methodological choices present further problems when considering the Breyer team’s attempts to model 100% RE scenarios for developing countries like Ghana, Nigeria, or Pakistan, where modelers should not even assume the suitability of existing road networks or supporting transmission for, say, wind project buildout in rural areas, let alone the existence of CO2 and natural gas pipelines. At a minimum, macro-level nodal studies like these must incorporate cost modifiers derived from more detailed operational frameworks to account for additional infrastructure not resolved by the simple model. Yet the LUT team does not consider these additional costs.
For perspective, consider that the Australian Energy Market Operator’s 2022 Integrated System Plan has estimated the country will require around 13,000 km of additional transmission and connection investments by 2050—this for a wealthy country of just 25 million people with an already mature electricity network. For populous developing countries with underdeveloped infrastructure and far tighter cost constraints, simple nodal models utterly fail to capture the required scale of network buildout. Some might argue that poor countries with limited existing infrastructure can reduce costs by building the most efficient possible power grid from the outset. However, given the sweeping demographic and migratory shifts that occur in the course of industrialization and economic development, it is equally if not more likely that planners will need to progressively reconfigure national infrastructure in response to evolving population and industrial centers.
1.6 Sensitivity testing and alternative scenario modeling are minimal or absent
LUT model papers from the Breyer team typically do not compare modeling results or costs for 100% RE scenarios against alternative scenarios, such as those with higher projected energy demand or ones that permit new nuclear power plant construction. This absence reflects a broader problem shared with many of the cited non-LUT model 100% RE Asia and Africa studies. Such sensitivity testing is required to robustly demonstrate that 100% RE systems are cost-efficient in more energy-intensive scenarios or relative to more technology-inclusive policy approaches.
For instance, the LUT model study covering West Africa arrives at quite low per capita electricity usage even for 2050, far below that for people in advanced economies. This contrast reflects a common tendency across climate and energy modeling to insufficiently account for future reductions in energy and wealth disparities between rich and poor countries. Nigeria, with a current population of 213 million and a projected 2050 population of up to 400 million, is assigned a nationwide annual electricity demand of 355 TWh in the study. This demand equals 0.9 to 1.7 TWh per million citizens, compared with American and Australian values of 12.8 TWh and 10.4 TWh, respectively, per million inhabitants. As such, future demand projections in the LUT analysis may vastly underestimate true system load in 2050, should poor countries today successfully achieve higher levels of electricity access to ensure a comfortable modern standard of living.
This model may in turn grossly understate the total system cost of a 100% RE system required to meet true future power demand, as capacity requirements scale with load. Given the temporally correlated nature of variable renewable generation, such total system costs could scale nonlinearly, driven by increasing storage requirements.
On the issue of cost, Breyer team papers often limit their discussion of cost-effectiveness to comparing their modeled 100% RE “total system” levelized cost of electricity (LCOE) to the LCOE of a new nuclear power plant, a theoretical gas plant with carbon capture and storage (CCS), or a theoretical coal plant with CCS (Figure 6). Or the 100% RE system LCOE is simply compared to a reported figure for the country or region’s current LCOE or the system LCOE of a “no policy” baseline scenario.
Figure 6: Similar excerpts from Breyer et al; Bogdanov et al.; Gulagi et al.; and Gulagi et al.
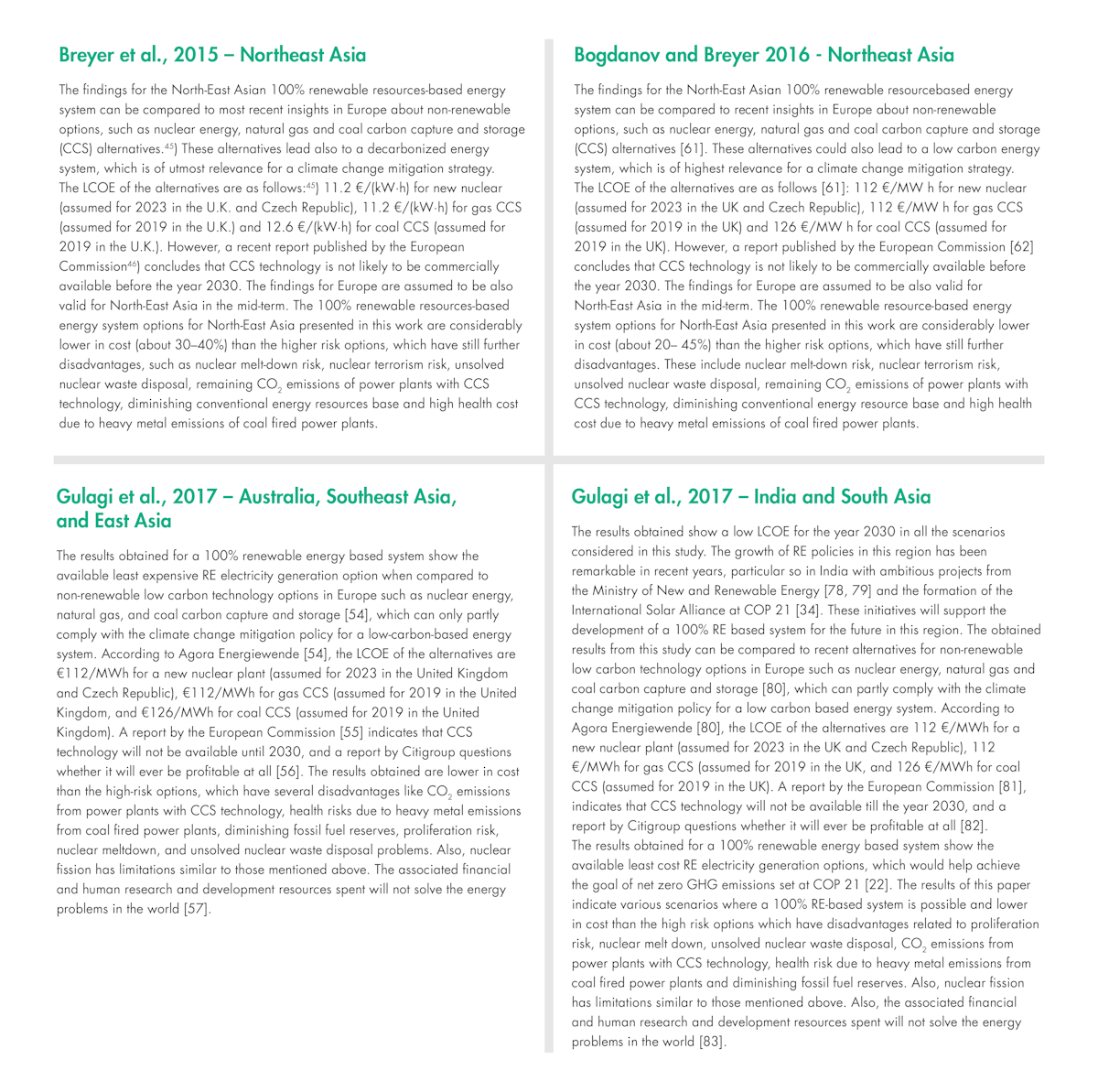
It is not valid to compare single power plant costs per kWh to system-wide costs per kWh. Such a comparison neglects the possibility that adding a few more expensive firm and/or dispatchable power plants to a modeled 100% RE system could replace significant renewables capacity, storage capacity, and transmission and distribution upgrades, thus helping reduce “total system” LCOE. Nor is the cost of entirely new nuclear power plants always the metric of relevance: costs to manage existing, already-built nuclear power plants to extend their operational lifetimes are considerably lower. Such lifetime extensions are a very relevant policy consideration for Asian countries operating large numbers of reactors like South Korea, China, and Japan.
Excluding nuclear energy from power sector decarbonization scenarios overlooks numerous useful aspects of nuclear technologies that could aid clean energy transition efforts:
- Affordable lifetime extensions of existing nuclear power plants.
- The possibility of lower nuclear power plant construction costs in some contexts, such as lower assessed costs for builds in China, Korea, or India.
- Lower-cost builds of new nuclear projects that add new reactors at existing nuclear power plants or repower retiring fossil power plants.
- Learning and cost improvements associated with successive builds of a given reactor design, particularly for small modular reactors that can take advantage of assembly line manufacturing.
- Molten salt reactors with integrated thermal energy storage that can operate at a high capacity factor while responding to variable renewable generation and capitalizing on electricity price arbitrage.
- Baseload nuclear units supporting industrial activities that require firm, reliable electricity and/or co-generated process heat and steam.
- Baseload nuclear power plants operating as part of poly-generation systems (potentially flexible production of electricity, steam, clean hydrogen, and other products).
- The potential for small reactors to compete economically with islanded renewables-based grids or expensive oil and diesel generation in remote and island communities.
Finally, LCOE values depend heavily on capital cost, operational lifetime, capacity factor, and numerous other assumptions and cannot be compared at face value without transparently providing—and ideally sensitivity testing—these underlying factors. Claimed system costs in LUT model papers become even less impressive when one recalls that the Breyer team assumes anomalously low renewable and storage technology costs for their model inputs. Realistic input costs are key variables in energy system modeling, and the LUT group’s use of unreasonably low cost assumptions makes it only natural for their 100% RE scenarios to produce attractively low “total system” costs.
1.7 Extensive methodological duplication exacerbates analytical limitations
LUT modeling papers exhibit little variation in their approach and methodology, compounding the impact of key assumptions and limitations. Many country-level LUT 100% RE studies show substantial duplication in study scope and approach, with successive papers covering Israel, Kazakhstan, India, and Iran published within a year or two of one another using very similar methodology. The second paper often adds only incremental value to the first. Given their sensitivity to the same modeling and methodological factors, these studies do not offer independent, separate evidence of 100% RE system feasibility for the countries of interest.
Certainly, striking similarities across many LUT papers seem to speak to the versatile and generalizable framework of the model. To extrapolate the findings to a new country, the researchers need only define a different simple grid network of nodes, adjust the solar and wind resource data and future energy load and sectoral assumptions, and hit run. Yet the LUT team’s recycling of regional and country studies, capital cost assumptions, and technology cost assumptions naturally begs the question of how much effort these studies are really putting into realistically tailoring their methodology for each area of interest.
As previous sections have shown, such concern is well warranted, with these dozens of similar LUT model studies all compounding fairly serious errors and questionable assumptions. While each paper in theory would present a new opportunity for peer review and iterative improvements in rigor, it appears that reviewers to date have not sufficiently pushed the LUT team to further refine their modeling approach.
In the LUT researchers’ broader review article cataloguing over 600 papers on 100% RE systems, the review’s authors themselves emphasize that a relatively small set of research groups are responsible for a large fraction of the listed studies. A closer examination of the subset of papers on Asia and Africa further indicates that this literature may not represent as comprehensive or definitive body of research as these hundreds of papers might imply.
2. Other Asia and Africa 100% RE studies are low quality
Figure 7: Categorization and evaluation of 25 and 61 studies cited by Khalili and Breyer
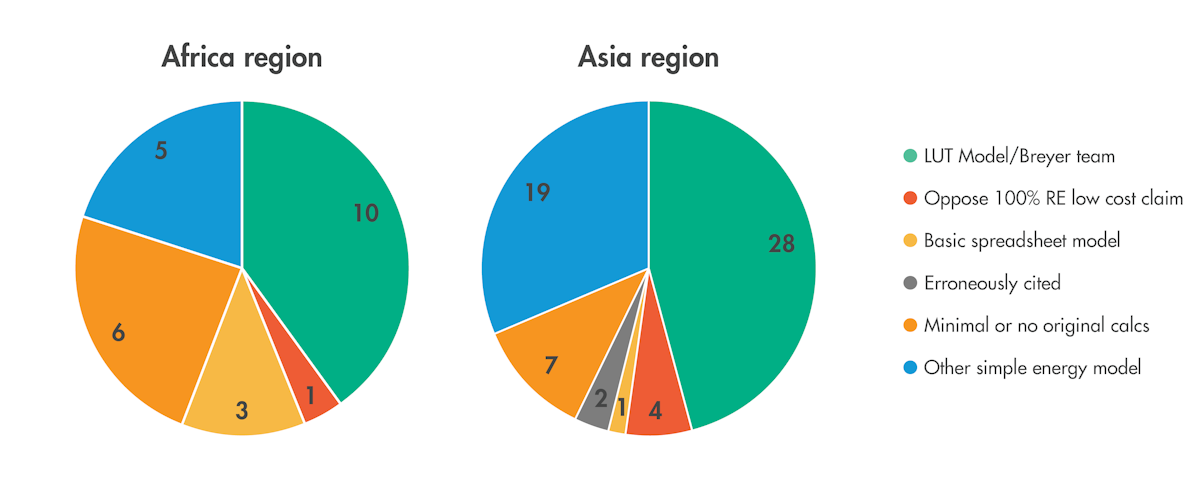
From these hundreds of 100% renewable system studies, the remaining subset of those covering Asia and Africa also requires important qualifications. The other 48 papers cited by Khalili and Breyer for these regions include papers of questionable quality, papers cited in error, papers that do not in fact model fully 100% renewable systems, and even some papers that find alternative scenarios boasting lower costs than fully renewable electricity grids (Figure 7). Generally, this problematic use of the literature underlines a dire need for higher quantity and quality of new energy systems modeling research with an Asia and Africa focus.
Click here for interactive table: ASSESSED 100% RENEWABLE LITERATURE FOR ASIA AND AFRICA
Of the remaining 48 Asia and Africa regional studies papers cited:
- 13 contain minimal or no original calculations at all, including some purely theoretical discussion papers, review papers describing other studies, and one extremely poor-quality paper using a “machine learning” model cited as evidence for 100% RE systems in Nigeria, South Africa, and Algeria.
- Five do not support the assertion that 100% RE systems are cost-effective (two studies find least-cost alternative scenarios that utilize nuclear or carbon capture, two do not fully decarbonize the power sector and thus are explicitly not 100% RE in scope, and one finds that 100% RE system scenarios for Japan increase total system costs by 80%-140%).
- Two papers employ only a simple spreadsheet version of the “renpass” energy system model (four citations, with one paper cited three times in the context of different countries).
- Two papers are erroneously cited. The sole country-level study cited for South Korea, for instance, is actually a study for Prince Edward Island in Canada. Another country-level paper said to be for Japan links to an article discussing the city of Altavilla Silentina, Italy.
- The remaining cited studies, mostly conducted in Asia, typically employ simple energy system modeling that cannot be used as a basis for power sector planning. Three studies, for instance, employ the simplified GENeSYS-MOD model, which only solves the future energy system at a reduced hourly temporal resolution. Many of the cited 100% RE system papers for Japan utilize simple nodal models with perfect lossless transmission.
- In general, the 100% RE literature for Africa is extremely sparse apart from LUT model papers, review/discussion papers, and poor-quality studies. The number of non-LUT simple energy system modeling papers for Africa can be counted on one hand (five papers), and none of these cover Sub-Saharan Africa at a detailed multi-country level.
The large historical review of hundreds of 100% RE studies sought primarily to catalogue articles “in which 100% renewable energy systems were surveyed,” rather than intending to include or exclude papers based on research quality or other criteria. As such, researchers, advocates, and policymakers must understand that this list includes literature reviews, papers containing only generic analysis, and even studies that find alternative scenarios to be more cost-optimal than 100% RE pathways. To characterize such research as overwhelming evidence supporting the feasibility and cost-effectiveness of renewables-and-storage-only energy systems would be to overstate what many of the cited researchers themselves might argue or claim.
3. How to make modeling better
How can future energy system modeling efforts better inform and advance power sector decarbonization efforts in Asia and Africa and elsewhere in the world? The LUT model studies and other papers based on simple modeling have significant shortcomings, while the overall literature covering Asia and Africa remains extremely thin. Above all else, the research community urgently needs to conduct many more studies examining energy systems across these regions.
Simple nodal models differ considerably from more detailed, realistic capacity expansion modeling that better highlights the economic and practical challenges facing fully 100% RE systems. Even realistic country-level capacity expansion models differ in important ways from the granular, high-resolution models that real-world grid operators use to assess power systems: such real-world models have highlighted operational difficulties that make 100% RE systems prohibitively impractical in many regional and national contexts. Simplified modeling can provide extremely useful insights, but it does not have the precision necessary to capture such important factors as additional infrastructure upgrades required to reliably operate a renewables-dominated smart grid in realistic electromagnetic transient simulations. At a minimum, simple macro-level models should incorporate modifiers, informed by more detailed studies, that seek to account for time, cost, and difficulty considerations that may emerge in higher-resolution assessments.
These factors matter. More complex modeling studies have often shown that including supporting nuclear, gas with carbon capture, or a minimal amount of peaking gas capacity could lower total system costs. But given the paucity of more detailed energy system modeling for Asia and Africa outside of developed East Asia, this discussion necessarily relies on the broader global literature, including studies focused on Europe and the United States.
Several distinct studies for the United States using two different models, for instance, found that existing and new nuclear and carbon capture plants can form part of the least-cost decarbonization pathway for the American power sector, particularly if solar and wind deployment is constrained (by, for instance, land availability, supply chain and project development bottlenecks, or political opposition). In contrast, 100% RE scenarios are possible, but typically exhibit higher total system costs. Meanwhile, allowing a model to deploy more versatile advanced nuclear reactor designs, such as molten salt reactors that utilize integrated thermal energy storage to dynamically ramp up and down, can encourage deployment of a significant number of advanced reactors alongside wind, solar, and storage capacity. A detailed regional study by New England’s Independent System Operator highlighted the cost-effectiveness of existing nuclear and backup gas capacity relative to scenarios that rely heavily on renewables-only strategies. France’s Réseau de Transport d’Electricité has found that new nuclear projects make economic sense and that achieving grid decarbonization is significantly easier with the help of existing and new nuclear capacity.
At least in the English language, high-resolution grid modeling studies for countries in Asia appear to be relatively sparse, with the notable exception of Japan, and seem nonexistent for Africa. At least five studies of Japan’s energy system have illustrated that existing nuclear power and deployment of CCS technologies could help reduce power grid decarbonization costs. Meanwhile, a number of simpler modeling studies exploring grid emissions reduction in China highlight the potential for considerable new nuclear power deployment.
For energy systems research and energy policy conversations to become more constructive and operationally relevant, researchers must construct and leverage more advanced modeling frameworks. Doing so can, in turn, help ensure that modeling studies are more useful for policymakers and planners facing real-world constraints.
To better inform planning efforts, future modeling analyses should strive to include:
- Robust scenario design and sensitivity testing that objectively evaluate the impact of adding or removing certain technologies and system constraints.
- Sub-hourly balancing and variable renewable generation.
- Spatially resolved capacity expansion modeling, including constraints associated with land suitability, transmission interconnections, CO2 pipelines, and gas infrastructure.
- Modeling of ancillary services and operational and planning reserve margins, considering in particular the impact of forecasting errors.
- Intra-temporal electricity price and total system cost analysis.
- Optimal power flow modeling of factors including but not limited to voltage and frequency control, short circuit level, and reactive power.
- System reliability assessment gauging infrastructure resilience to disasters, equipment failure, and similar contingencies—both singular events and common cause events.
Christian Breyer and his fellow 100% RE researchers have themselves called for more advanced energy system modeling of this nature as the next, necessary development for the 100% RE systems literature. Thus, both critics and proponents of the existing 100% RE research appear to agree that a shift toward more rigorous, high-resolution, operationally complex modeling efforts will improve the overall quality of academic and policy discussions and enhance our practical understanding of how to efficiently develop decarbonized power grids.
4. Conclusion
To point out the limitations of these dozens of 100% RE system studies should by no means be interpreted as arguing that these papers fail to make any useful or positive contribution to climate and clean energy policy. Simple energy system models serve important purposes, including potential validation of key first-order considerations. The problem is that environmental advocates and policymakers—and some researchers—often go on to leverage such research in support of far more aggressive claims.
“This research clearly indicates that a 100% renewable resources-based energy system is THE real policy option.” Christian Breyer and his coauthors’ declaration was unambiguous and adamant in the abstract of their 2015 paper alleging the feasibility and cost-effectiveness of a 100% RE system spanning from China and Mongolia to Japan and South Korea. Breyer’s team’s more recent review of 100% RE system studies likewise leaves no room for argument: “The main conclusion of most of these studies is that 100% renewables is feasible worldwide at low cost.” Mark Jacobson, one of Breyer’s coauthors, claimed in a recent interview that the review demonstrates “we can go to 100% renewables in the electric power sector or all sectors with just clean renewable energy. No nuclear power. No fossil fuels. No carbon capture.”
But dozens of these 100% RE studies for Asia and Africa are not only highly simplistic, but deeply flawed. At best, these analyses only establish that fully renewable grids do not fail basic sanity tests of their feasibility, but they do so in extremely streamlined models that assume away many of the operational challenges that a 100% renewables system would face in practice. Much of the 100% RE literature for Asia and Africa in particular originates from a single team at one university, whose studies adopt highly questionable cost and technology assumptions.
No valid energy system modeling effort could assert that renewable energy project capital costs in Sub-Saharan Africa today will be on par with the cost of projects in China or that synthesizing methane from direct air CO2 capture and electrolytic hydrogen is a remotely realistic technology option that can help Africans decarbonize much of their continent’s electricity sector within a decade. Such assumptions grossly misrepresent the true scale of the challenges facing Asian and African peoples who account for much of the world’s population, reside disproportionately in low- and middle-income countries, and must simultaneously balance clean energy deployment with critically important human and economic development priorities.
In Asia, civil society groups have seized on the 100% RE literature in support of their renewables-only policy platforms, with the LUT team’s research directly influencing national energy policy debates. International environmental NGOs, including Greenpeace International, Climate Analytics, Energy Watch Group, and Friends of the Earth, regularly cite the 100% RE literature to call for exclusion of nuclear power from energy policies worldwide. Policy recommendations recently presented by Japanese environmental nonprofit Climate Integrate call for a national ban on new nuclear construction, suggest even existing nuclear capacity might be more expensive than renewables, and call for renewable synthetic methane as part of their future energy vision for Japan. Christian Breyer and his LUT team have directly collaborated with the Renewable Energy Institute, a Japanese think tank, to produce reports alleging that new renewable projects will economically outcompete even existing nuclear capacity by mid-decade. The LUT team has also worked with the Taiwanese nonprofit Industrial Technology Research Institute to produce power sector decarbonization pathways for Taiwan that support a nationwide nuclear phaseout.
Meanwhile, many discussions on the future of renewables in Africa reference the 100% RE literature to argue that the continent’s clean energy transition is close at hand. A 2022 report from the African Development Bank points to LUT team research, noting approvingly that “a well-designed Africa-wide clean energy portfolio with around 80 percent generation from solar PV and wind that incorporates all five options could meet Africa’s entire electricity demand for every hour of the year at an overall levelized cost of electricity of roughly $0.06 per kWh.” British International Investment, the UK’s development finance institution, to justify its exclusion of investment in most fossil energy projects, similarly cites several LUT team papers as evidence that renewables-dominated energy systems in Africa could lower electricity costs. Recent reports from IRENA, Climate Analytics, the National Business Initiative and World Wildlife Fund, and the Columbia Center on Sustainable Investment uncritically reference the LUT team in articulating green investment recommendations for Africa. The well-known Climate Action Tracker program directly utilizes LUT papers to define sectoral energy policy benchmarks.
This narrow and analytically flawed body of research thus carries real-world implications, with real-world consequences. Clearly, the international research community must urgently prioritize more rigorous energy system modeling studies covering Asia and Africa, using both simple and more advanced, high-resolution approaches. To best inform energy policy and planning decisions, stakeholders in Asia and Africa will need to have and use a much wider and more robust body of competing research moving forward.
In the meantime, 100% renewables rhetoric has imposed an awkward dynamic on energy policy discussions, in which many climate advocates and members of the public mistakenly think that it is common, accepted knowledge that a fully renewable grid is cheap and readily achievable starting today, worldwide. In practice, technologies like nuclear power and carbon capture represent useful tools in the broader low-carbon toolbox that climate advocates would be prudent to evaluate with an open mind, appreciating their potential to enhance energy system reliability and attenuate future total system costs. Meanwhile, all clean energy technologies remain unacceptably inaccessible for low-income countries, given financing barriers, higher costs, infrastructure limitations, and numerous other factors.
African and Asian policymakers should carefully examine 100% RE systems research for unspoken, underlying expectations that Ethiopia, the Democratic Republic of the Congo, or Mongolia should achieve economic development through the pure path of renewables and storage alone, without any nuclear power, fossil fuels, or even large dams. Rather than entertain the possibility that such a path might not be in the interests of African and Asian peoples, some researchers seem eager to skew methodology and assumptions to produce results that align with such aesthetic preferences.
For their part, climate advocates must recognize the clear truth that affordable power sector decarbonization still remains well out of the reach of low-income countries in Sub-Saharan Africa and Asia. The world has no right to ask the global poor to choose climate goals over accessible, affordable electricity. Rich countries must therefore support rapid universal electrification across the Global South using an all-of-the-above approach that permits pursuit of fossil fuel power plants alongside renewables, hydroelectricity, nuclear power, and carbon capture. Developed countries, meanwhile, have the duty to redouble their own climate efforts and bring the cost of clean technologies down further, while opening up more carbon space for developing countries to achieve higher living standards.
Acknowledgements: Ben Heard. Auke Hoekstra strongly disagreed with the first draft of this article but provided detailed criticism for which we are grateful.