What is a Nuclear Reactor with Thermal Energy Storage?
And why a utility, or its customers, would like one.
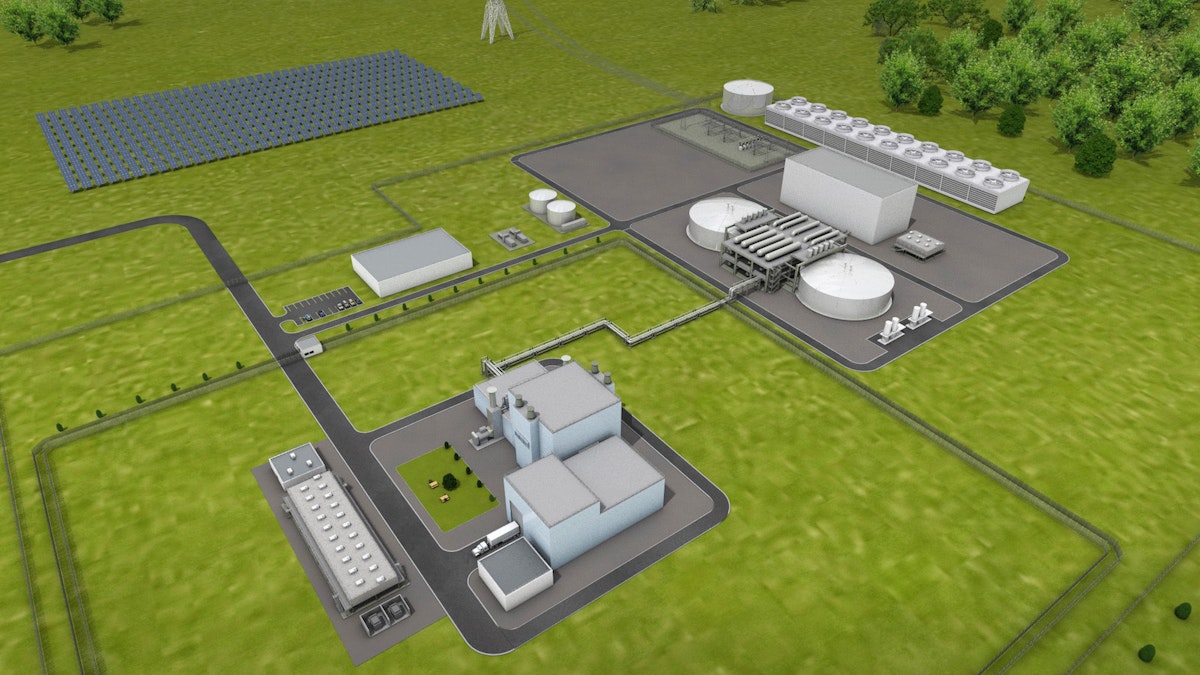
-
-
Share
-
Share via Twitter -
Share via Facebook -
Share via Email
-
The hard part of a carbon-free electricity system isn’t making carbon-free electricity. It’s matching the electricity generated to demand every minute, of every day, of every season.
Utilities do that now by burning fossil gas, a process that can easily be ramped up or down as conditions demand. But that is unlikely to be an option in a zero-carbon economy.
Better, more advanced energy storage has been widely recognized as a way to make better use of unscheduled production from wind and solar farms, which is out of sync with customer demand. Production of energy from nuclear power plants can be scheduled, but reactors work better if they can produce energy 24/7, so storage at a reactor helps nuclear keep running while storing up energy so it can fill in the gaps in a system that makes use of a lot of wind and solar.
A special kind of storage, of heat instead of electrons, is emerging as one promising, cost-effective option. And the best way to charge up a heat storage system is with a nuclear reactor. Hence, the Advanced Reactor with Thermal Energy Storage (ARTES).
How do you store heat?
Heat storage comes in many different forms. It may be a tank full of rocks, with a liquid or gas circulated inside to move heat in and out. It can also be a few hundred thousand gallons of molten salt.
This is not what most people think of when they hear “batteries.” The AAs you put in a TV remote are chemical batteries with positive and negative terminals. These types of batteries seem simple, and do a good job of storing small amounts of electricity, but they are made of scarce materials that are environmentally damaging to extract. They are also so expensive that they may be cost-effective only if they get very frequent use, being charged and discharged daily, or perhaps several times a day. They also tend to wear out in a few years.
A heat battery is far simpler and less expensive.
To understand how, remember that heat is an intermediate step in the production of electricity. Heat, from a nuclear reactor, from a coal-fired furnace or a gas burner, is used to boil water into steam, and then the steam is used to spin a turbine, which turns a generator and makes an electric current.
An ARTES plant would do the same, but not all at once. A reactor producing a steady flow of heat could charge up a heat battery on a summer morning when electricity demand is low. The ARTES plant operator could then tap that stored heat to make electricity in the late afternoon and early evening, when electricity demand peaks and when other kinds of production, like solar, are low or offline.
Why can’t solar and wind use heat batteries?
They could, but it’s a roundabout, inefficient way to supply carbon-free electricity. Solar and wind make electricity without going through a heat-to-steam cycle. A solar or wind farm operator could use their electricity to heat up some storage medium, for conversion back into steam and then electricity when the energy is needed. But it’s not as efficient as using a source that makes heat in the first place.
Some solar and wind farms do use chemical batteries. A particularly effective use is for very short-term storage, for seconds or minutes, which allows them to produce electricity at a steadier rate. Smooth production profiles are more helpful to the grid. Chemical batteries are very good at switching from charge to discharge and back almost instantly.
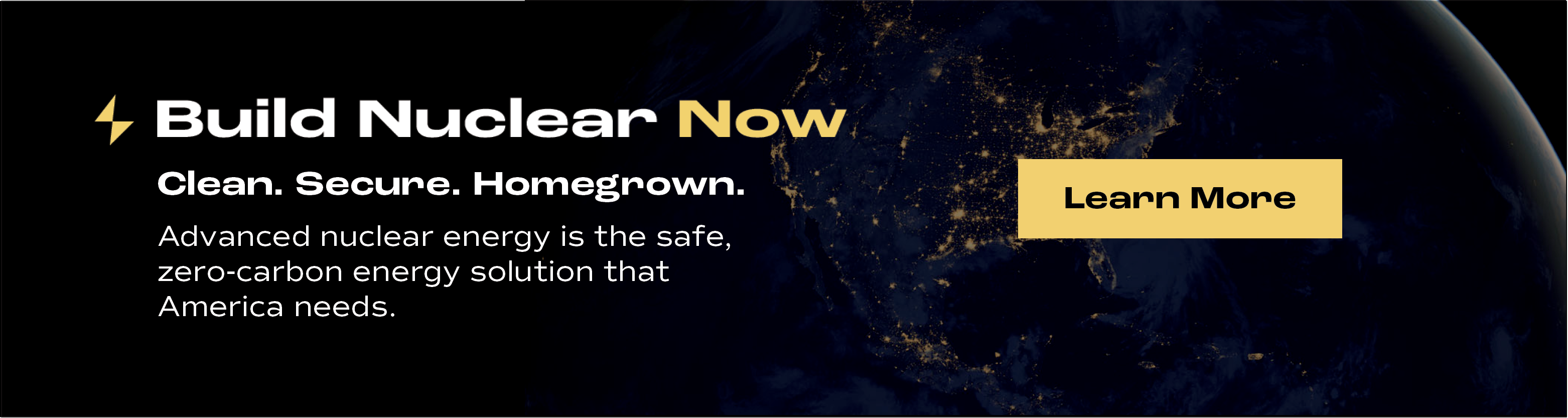
The chemical batteries on solar or wind farms can typically store a few minutes or a few hours of production, but these are costly. They also raise the carbon footprint of the kilowatt-hours delivered, because the batteries return only part of the energy that is used to charge them. (The rest is lost as low-level heat.) And the manufacture of the batteries has a substantial carbon footprint.
Are there financial benefits to heat batteries?
Yes. In most of the United States, the wholesale price of electricity closely tracks the balance of supply and demand. In places with a lot of renewable energy installations, the prices can fall to zero, or even less than zero, when those sources saturate the power grid, which generally happens during periods of relatively low demand.
Stated in financial terms, ARTES lets a nuclear reactor save up its production until prices are high, and avoid selling a lot of power into a market that has already crashed from overproduction. It also lets a reactor owner run the machine continuously at full power, which is important because reactors are expensive, and a key to making them successful in the competitive marketplace is keeping them in full use all the time. And in some reactor designs, operating at a steady state means less wear-and-tear on the fuel and other components, compared to raising and lowering production over the course of the day.
(The parts of an ARTES plant that would not run at full power 24/7, notably the steam turbine and the electric generator, are relatively inexpensive. So is the heat storage.)
Seen from the consumers’ point of view, ARTES is shaving the peak price, by delivering electricity at hours when renewable sources are in short supply, or completely unavailable. From an environmental point of view, it turns a carbon-free reactor into an explicit complement for variable wind and solar.
What is the status of ARTES today?
Under the Department of Energy’s Advanced Reactor Demonstration Program, one of the two projects that is supposed to produce a commercial demonstration of an advanced model later in this decade is an ARTES unit. It will use a 345-megawatt reactor to heat up tanks of molten salt; on the other side of the tanks, steam generators will use the salt’s heat to boil water and spin turbines, producing anywhere from 100 megawatts to 500 megawatts, depending on grid demand.
The project is a partnership between GE-Hitachi, which has an advanced design for a high-temperature reactor, and TerraPower, a company founded by Bill Gates and other investors. It will be built in Kemmerer, Wyoming, on the site of a coal plant that is retiring, and will re-use some of the coal plant’s infrastructure, and employ some of its workers.
Other reactor designs, further from commercial deployment, use a large volume of molten salt in the reactor vessel itself, and can operate over a wide temperature range, so that they can be tapped for electricity at variable rates, as grid conditions require.
Storage at a reactor could solve the two key problems for wind and solar. First is “curtailment,” which means having to unplug the generators when there is no market for the energy. The second is leaving customers high and dry when the sun goes down, or the wind stops. If a reactor can direct its production to heat storage, it makes space on the grid for peaks of production from sun or wind, making the best use of those assets. And the storage provides crucial reliability for a grid from which the fossil fuels have been purged. That means a lower-cost route to a clean energy system.