Atoms and Influence
What’s Driving Innovation in Nuclear Energy?
-
-
Share
-
Share via Twitter -
Share via Facebook -
Share via Email
-
When POWER magazine, where I am an editor, launched its first issue in 1882, the world was only beginning to grasp how electricity, a new and versatile form of energy, could revolutionize human life. Since then, the magazine’s pages have reflected the rapid evolution of the technologies and markets that have characterized the world’s power sector.
Yet a recent attempt by the magazine’s editors to parse that evolution into a clear chronology—from the invention of the light bulb through today’s nuclear influencers, such as Isabelle Boemeke, or Isodope, as she’s known on TikTok—proved nearly impossible.
The assignment set out to capture the power sector’s most significant breakthroughs in the hopes of providing historical context for today’s energy transition. But what it revealed was an unruly treasury of technology breakthroughs fueled by policy intervention, environmental and safety concerns, shifting market expectations, and ambitious ideas. Attempts to even organize those tales into a particular thread—for example, the history of steam, or coal, or natural gas, or hydrogen—was just as tangled, always driven by pressures and tumult within any number of interconnected areas.
And while every one of the 13 decades that POWER magazine has been in print has been definitive for electricity generation technology, policy, and business in some significant way, few have been as transformative—or as jumbled—as the last. The power industry has grappled with multiple disruptions, including decentralization and electrification, a boost in shale gas production, a proliferation of renewable power and battery storage, an increasing embrace of digitization, and greater consumer interest in—and concern about—where their power comes from.
Each one of those developments has been complicated and nuanced in its own right—to say nothing of the myriad ways in which they’ve combined.
Even so, it is important to try to zoom out from time to time, and especially when it comes to nuclear energy, which is today enjoying a revival championed by grassroots climate advocacy, including the new nuclear influencers, who focus solely on the benefits of reactors. Their work may push nuclear energy some way toward social acceptance, but if my colleagues and I have learned anything of late, it is that only by finding where all the threads of all the energy stories weave together—and where they leave gaps—can we identify the opportunities to push innovation and collaboration further.
A History of Breakthroughs
Innovation in other sciences has driven a good chunk of nuclear energy’s history. Although the concept of the atom was fairly well-developed when POWER released its first issue, scientists had not yet figured out how to harness the energy contained in atoms. Just 13 years later, though, in 1895, the accidental discovery of X-rays by German engineer Wilhelm Röntgen started a wave of experimentation in the atomic field. Close on his heels came French physicist Antoine Henri Becquerel, who discovered radiation. Then Marie and Pierre Curie conducted their radiation research and coined the term “radioactivity.” Ernest Rutherford, a New Zealand-born British physicist who many consider the father of nuclear science, postulated the structure of the atom, proposed the laws of radioactive decay, and conducted groundbreaking research into the transmutation of elements.
In the decades that followed, with a base understanding of atomic principles established, wilder discoveries took center stage. In 1934, Italian physicist Enrico Fermi showed that neutrons could split atoms. Two German scientists—Otto Hahn and Fritz Strassman—expanded on that knowledge in 1938 when they discovered fission. Scientists then turned their attention to the puzzle of developing a self-sustaining nuclear chain reaction. In December 1942, a team led by Fermi demonstrated the world’s first nuclear reactor, Chicago Pile-1, transforming scientific theory into technological reality. And so the world was launched into the nuclear age.
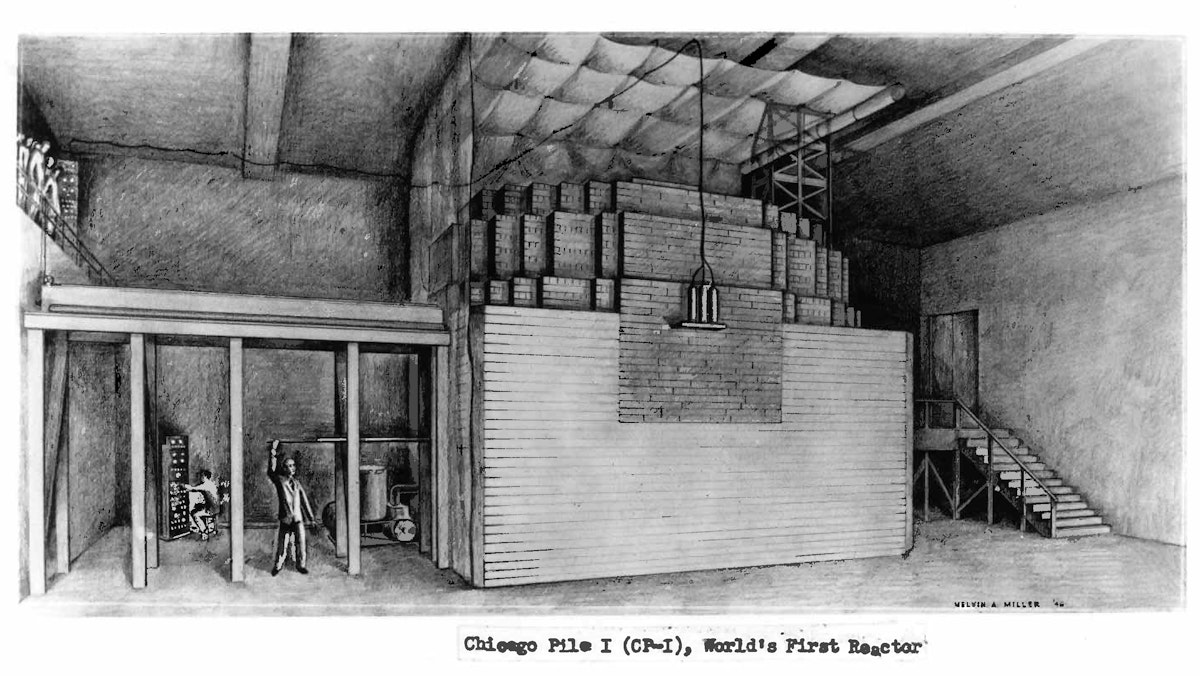
From there, most early atomic research focused on developing high-power reactors to produce plutonium fuel for nuclear explosives as part of the Manhattan Project. But after the end of World War II and the deployment of the first nuclear weapon, Congress passed the Atomic Energy Act of 1946, which transferred the development and production of atomic weapons—as well as budding research and development projects for civilian uses of nuclear energy—to the civilian Atomic Energy Commission (AEC).
The same year, the Manhattan Project began developing a pilot “pile” or plant for nuclear power at Oak Ridge, Tennessee, intending to harvest heat energy at a conventional central station for steam electric generation. And in 1947, the AEC proposed and funded four new reactors that would make use of enriched uranium, which was then newly available: a fast reactor to explore the fuel “breeding” possibilities; a navy thermal reactor that would serve as a prototype for submarine propulsion (now known as STR or S1W); a materials testing reactor; and the Knolls intermediate reactor.
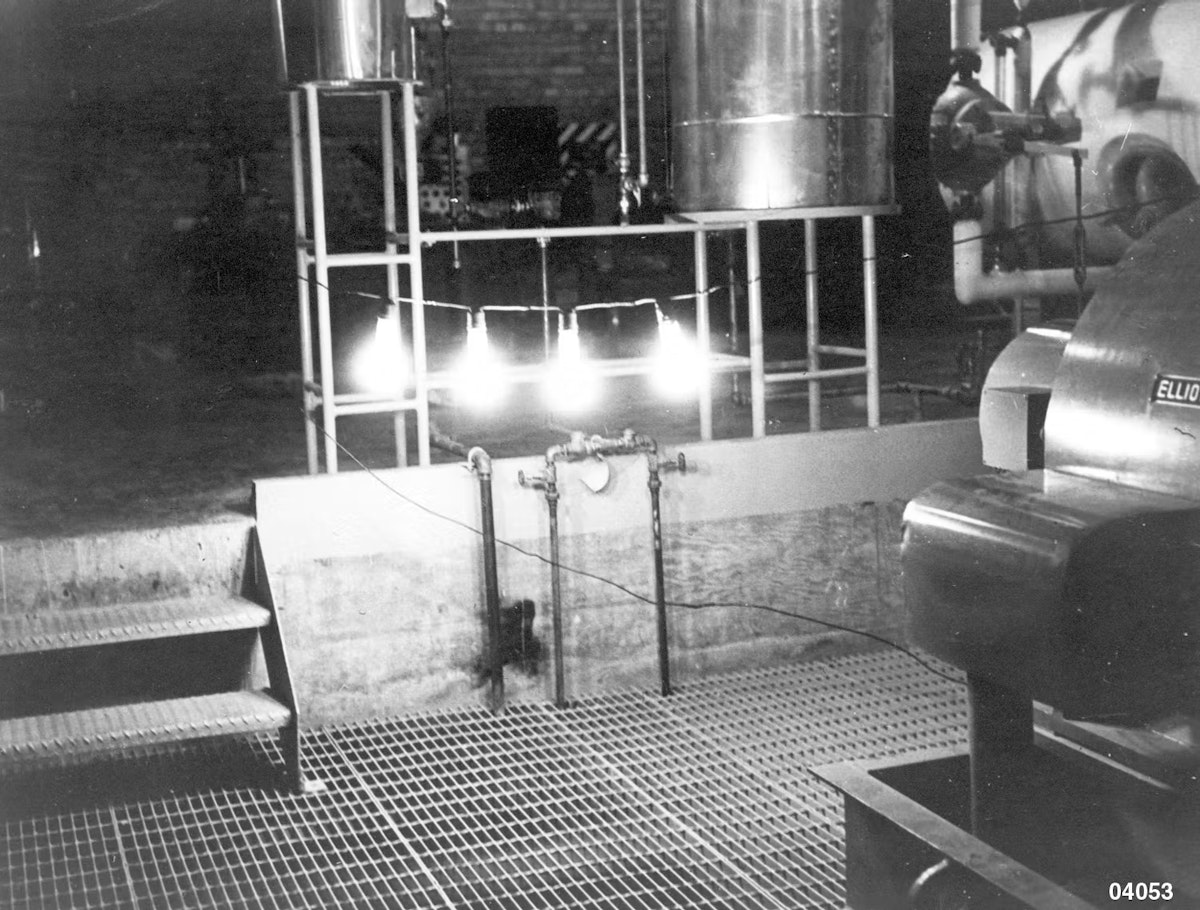
Three were developed at the National Reactor Testing Station (now Idaho National Laboratory, or INL), which was established in 1949 on an 890-square-mile desert site. Its mission was to develop civilian and defense nuclear reactor technologies and manage spent nuclear fuel. Over its long history, INL, now a U.S. Department of Energy laboratory, has hosted 52 pioneering nuclear reactor projects. Its first, Experimental Breeder Reactor-1, in December 1951 became the first reactor to generate usable amounts of electricity.
Or, at least, that is the most straightforward and linear plotting of the story.
Nuclear Ideals Meet Geopolitical and Economic Realities
Of course, the real story of nuclear can’t be told without the race between the United States and Soviet Union to establish domestic nuclear defense capabilities while also cornering the market on nuclear energy. In the Soviet Union, scientists modified an existing graphite-moderated channel-type plutonium production reactor for heat and electricity generation. In June 1954, that unit, located in Obninsk, began producing electricity. A few years later, in 1957, the first commercial U.S. nuclear power plant—Shippingport Atomic Power Station, a light-water reactor (LWR) with a 60-MW capacity—was synchronized to the power grid in Pennsylvania.
While Shippingport’s success marked a major starting point for the commercial viability of nuclear power in the United States, nuclear’s adoption, as with other early power technology designs, stemmed partly from unanticipated events and some path dependency. Shippingport's most prominent legacy was perhaps to deliver a stronghold for the LWR, which remains the most widely used design of nuclear power generation.
LWRs use ordinary water as both the coolant and the moderator for a controlled nuclear reaction. The technology is split into two basic types: pressurized-water reactors (PWRs), which keep water under high pressure and achieve high temperatures without boiling in the primary system, and boiling-water reactors (BWRs), which generate steam directly. Both types produce steam to drive power-generating turbines.
Some power industry historians posit that the LWR triumphed over several other reactor designs because of technical and economic advantages. General Electric, for example, spearheaded the commercialization of the BWR because the design was seen as the least risky available alternative. But initial decisions set the playing field for later ones.
To encourage investment in the costly new technology by risk-averse utilities, the AEC subsidized an extended a series of demonstrations between 1953 and 1960 under the Power Reactor Demonstration Program (PRDP). While the program included reactor types other than LWRs, the PRDP did not provide sufficient assurances about cost competitiveness for any nuclear models, which led to a period in which the government subsidized construction and demonstration. That included underwriting research and development and design costs, government ownership of the reactor, and fuel allowance clauses.
Cost concerns prompted General Electric and Westinghouse to offer reactors with larger generating capacities. Initial acceptance, starting with the Oyster Creek plant in New Jersey, eventually laid the foundation for the first turnkey plant contracts—characterized by vendor offers to construct and guarantee plant operability at a fixed price.
Critical to these sales were assumptions that nuclear plants could compete with fossil fuel plant costs at the time, but by 1966, when the first 25 nuclear plants had been deployed, capital cost investments were still roughly twice what they had been estimated when purchased.
That looked like trouble for nuclear, but then came a sharp surge in coal prices later in the 1960s, alongside the establishment of a concerted and expanding grassroots environmental movement. By the early 1970s, nuclear innovation began a ramp-up to meet market demand for fuel diversification. Fuel diversification during and after the oil crisis in 1973 offered another lucrative avenue for nuclear. Through it all, accidents—prominently, Three Mile Island in 1979, Chernobyl in 1986, and Fukushima in 2011—reshaped design innovation, spurring safer designs for reactor and fuel technology.
Nuclear Nationalism
Around the world, as in the United States, a healthy dose of scientific nationalism was overlaid on the economic factors driving (or stalling) nuclear innovation, particularly in the United Kingdom, France, Germany, and Canada. Domestic designs still comprise the bulk of these countries’ nuclear fleets. Canada, notably, chose to pursue a single design, a version of a pressurized heavy-water reactor (PHWR) called the CANDU (Canadian Deuterium Uranium), owing to the country’s abundance of uranium but lack of uranium enrichment resources. The conservative technical approach paid off in later years, given major changes in world fuel economies that made PHWR costs attractive and because CANDU fuel did not require reprocessing.
Meanwhile, technology uptake in several other countries evolved from technology transfer from vendors abroad into domestic development. That includes PWRs in France, Japan, South Korea, and China and BWRs in Japan. In these countries, governmental drive and international collaboration proved essential for the success of nuclear development. How future generations of reactors, which promise to be highly economical, incorporate enhanced safety, are digitally capable, produce minimal waste, and are resistant to proliferation, will fare will depend on a number of emerging factors, including economics, market viability, and application.
According to the International Atomic Energy Agency (IAEA), 437 nuclear power reactors, a combined 389.5 GWe, were in operation at the end of 2021—making up around 11% of the world’s global installed power capacity. The world’s fleet mainly comprised pressurized-water reactors (PWRs), boiling-water reactors (BWRs), pressurized heavy-water reactors (PHWRs), and Soviet light-water gas reactors (LWGRs). A smaller fraction of the world’s fleet comprises gas-cooled reactors (GCRs) and fast reactors. High-temperature gas reactors (HTGRs) have been under development since 1947, and though a handful have commercially operated, only one is currently in trial operation in China.
Innovation Is a Fine Balance
Today, innovation in the nuclear industry relies on a complex set of factors—many of them unchanged since nuclear power’s inception 80 years ago.
The nuclear industry is still a vast amalgam of utilities and power plant operators, R&D performers, regulators, technology vendors, and fuel providers. Over the past few years, this set of key players has absorbed policymakers, as well as the rapidly expanding realm of climate change advocacy and, most recently, influencers.
Since 2020, a consensus has solidified around the idea that energy-related decarbonization cannot occur without nuclear energy. But boosting nuclear is easier said than done, since decisions affecting nuclear innovation intermingle climate goals, technological advancement, legislation, makeup of the existing power structure, domestic concerns, and national ambitions, including prestige, national security, and energy independence.
Policymaking has to think of all that, and rely on laborious policy direction, including sound visioning and planning practices and proper evaluation and oversight mechanisms. Policymaking may also bear on nuclear legal frameworks, which include regulatory constraints related to health and safety regulations.
These influences are then laid over what some in the power industry deem the “realistic” drivers of innovation, such as market demand, which all power-generating technologies have in some way responded to since the birth of the electric generating industry.
In the United States, power companies and utilities have played an outsize role in bolstering demonstration or supporting nuclear technology advancement. While power company financial outcomes have historically determined where investments are prudent, the sector is currently grappling with a long list of energy transition–related risks, foremost among them reliability, resilience, and environmental compliance.
Ultimately, however, innovation driven by the power sector also relies on the availability of qualified personnel and access to research and development facilities and knowledge. It thrives on organizational culture for innovation. While aligning these factors won’t likely be immediate, change is undoubtedly afoot.
Driven by the urgency of keeping the nuclear sector relevant in the push for decarbonization, the industry’s biggest asset today may be multiarmed campaigns to underscore the power resource’s role within a larger, and rapidly changing, power system and society.
Messaging that has mostly highlighted the inevitable reliance on fossil resources if nuclear plants are shuttered and that mainly underscores nuclear power’s carbon-free and reliability benefits has not done much to push the needle. Of course, these messages are not wrong, but by focusing on one narrow dynamic within the power system, they miss the complexity behind what’s gone right—and wrong—so far. After all, power is a terribly complex integration of resources, processes, and output.
Ensuring nuclear’s future will thus require appealing not just to needs in the moment but recognizing nuclear energy’s complementary contributions for the long term. That includes its capabilities for more agile and flexible power, its applications for industrial heat production, its benefits for industrial electrification, the promise of nuclear hybridization with energy storage and renewables, and even the possibility of distributed energy from “micro” reactors.
All this is perhaps too much for one spokesperson, influencer, or chronology. But it is the truth.