What New Net-Zero Studies Tell Us About Electricity Decarbonization
-
-
Share
-
Share via Twitter -
Share via Facebook -
Share via Email
-
There is a view among some in the environmental community that “any solution that doesn’t show up in the next 10–20 years is essentially no solution at all.” They suggest that we have all the technologies we need to decarbonize today, and that all we lack is the political will to do so. Clean energy technologies like wind and solar have fallen dramatically in price in recent years, and will likely provide the bulk of new clean energy for the US over the next decade or two.
At the same time, however, these technologies cannot effectively decarbonize the entire economy — or even the power sector — alone. New state-of-the-art energy system models suggest that the current decade is a critical time in which we need to both accelerate the deployment of existing clean energy technologies and heavily invest in RD&D for maturing and improving a range of technologies that will be needed longer-term — such as advanced nuclear, gas with carbon capture and storage, enhanced geothermal, blue/green hydrogen, and direct air capture.
A slew of new net-zero studies have been published in recent months, including Princeton's Net Zero America (NZA) project, the Vibrant Clean Energy Zero By Fifty scenario, and by a team of researchers led by Jim Williams at USF. All three of these take a deep-dive into how the US could reach net-zero emissions by 2050, down to the level of where each new generating facility might be located, where new transmission lines would be built, and how electricity generation sources can meet hourly grid demand in different regions of the country. Each study contains multiple scenarios looking at the sensitivity to future technology prices, land use constraints, and other factors. But for simplicity, we focus in this comparison on their marker scenarios: E+ for NZA, the default Zero By Fifty scenario from Vibrant, and the central scenario from Williams et al. Both NZA and Williams et al. use a combination of the EnergyPATHWAYS (EP) and RIO models to generate their scenarios, while Vibrant uses their WIS:dom model.
While the models differ in important ways, they all paint a broadly similar picture. Wind and solar expand rapidly in the next three decades. US coal use falls off a cliff, reaching zero by 2030 or 2035. At the same time, natural gas use stays rather flat — or even increases modestly — between 2020 and 2030, as it serves a key role in filling in the gaps in variable renewable generation. Gas capacity actually increases in two of the three decarbonization models through 2050, though capacity factors — how often the gas plants are run — fall rapidly, and gas increasingly becomes a blend of hydrogen and methane closer to 2050.
The figure below shows the current 2020 US electricity generation mix, as well as the projected generation mix in 2030, 2040, and 2050 across each of the three models.
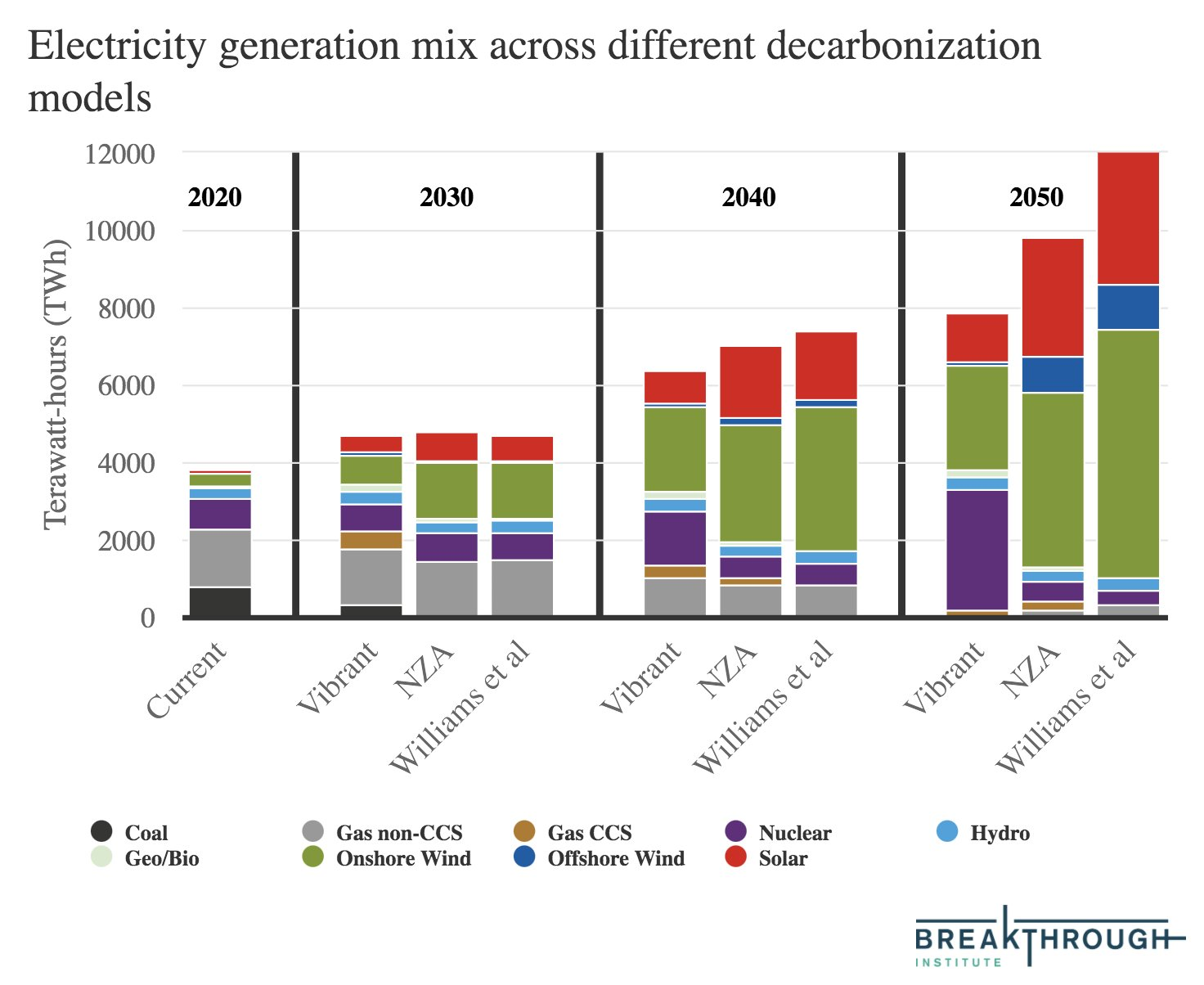
A few things immediately stand out:
First, there are fairly sizable differences in future electricity demand; in all cases, it is well above 2020 levels, with 2050 generation roughly twice current levels in Vibrant’s model, 2.5x current levels in NZA, and more than three times current levels in Williams et al. These differences in overall demand presumably arise from differing assumptions around economy-wide electrification rates, demand, and future energy efficiency. The degree to which other sectors of the economy can be effectively electrified will also impact the shape of demand curves and the types of generation that are best-suited to meet demand; both NZA (E-) and Williams et al. (Delayed Electrification) examine scenarios where electrification is more difficult to achieve.
Second, future generation looks quite different between the Vibrant WIS:dom model and the EP/RIO-based results from NZA and Williams et al. While variable renewables — wind and solar — supply the majority of generation in all three, Vibrant’s model sees a big expansion of nuclear — in the form of small and modular reactors and molten salt reactors — starting in the mid-2030s. Both NZA and Williams et al. expect offshore wind to play a major role in the 2040s, while the Vibrant model has relatively modest offshore wind generation.
Finally, while NZA and Williams et al. have some remaining gas generation in 2050 (some with CCS, some blended with H2 and offset by direct air capture), Vibrant has only a small amount of gas with CCS. As mentioned earlier, both NZA and Williams et al. have variations of their model with different assumptions around future technology prices, constraints, and land availability. In some scenarios, nuclear and CCS play a relatively larger role, while other scenarios have an even larger role for variable renewables.
Variable renewable energy provides between 51% and 91% of total electricity generation by 2050 across the three models — though in all cases a sizable chunk of clean firm generation remains needed (as well a massive expansion of transmission capacity, battery storage, and other complementary technologies). The figure below shows how the three models expect generation from firm and variable sources to evolve over time.
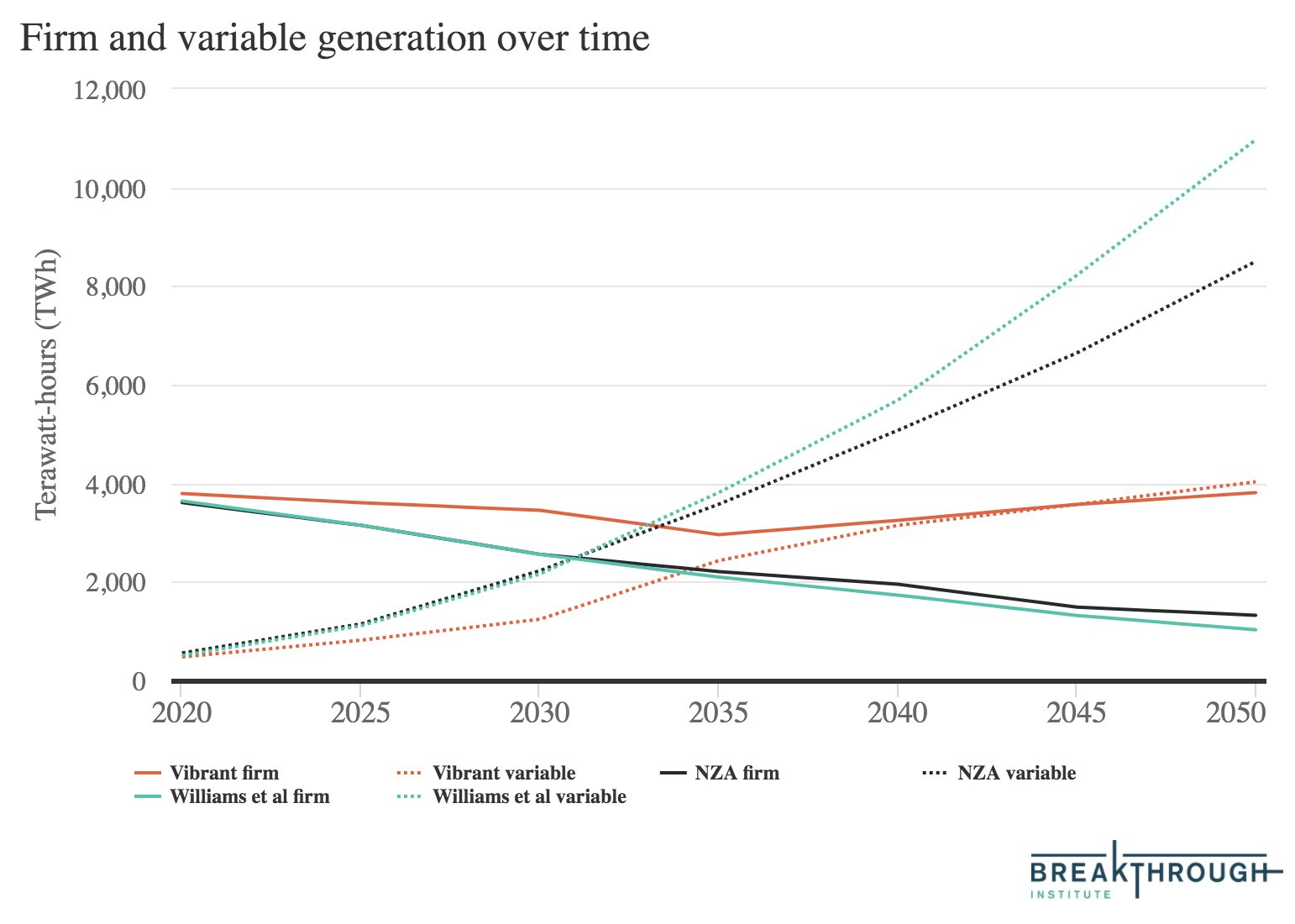
Here firm generation includes both fossil sources like coal and gas without CCS as well as clean firm generation from hydro, geothermal, biomass, nuclear, and gas with CCS. Variable generation includes solar, onshore wind, and offshore wind. In all scenarios, variable generation dramatically increases while firm generation plateaus or gradually declines. Vibrant’s scenario is notably different from the other two, as its firm capacity remains high in 2050 — with next-generation nuclear technology largely replacing gas and traditional nuclear. In Vibrant’s scenario, molten salt reactors provide firm, always-on generation, while small and modular nuclear reactors replace the load-following capabilities of natural gas today. However, the other two studies also maintain notable amounts of firm clean generation.
All three scenarios also feature large-scale expansion of transmission, energy storage, and demand management to help support higher levels of intermittent generation. They demonstrate that the supporting technologies around renewable energy are in many ways just as important as the renewable energy itself. All of these scenarios also involve decidedly non-trivial changes in land-use. For example, the NZA model has approximately 8% of the US land area to be devoted to energy production (excluding new transmission) in its E+ case. The figure below shows an example of how land would be used around the St. Louis area in the NZA scenario — though note that in the case of wind farms, the vast majority of the land area (barring the turbine footprint) can simultaneously be used for agricultural operations.
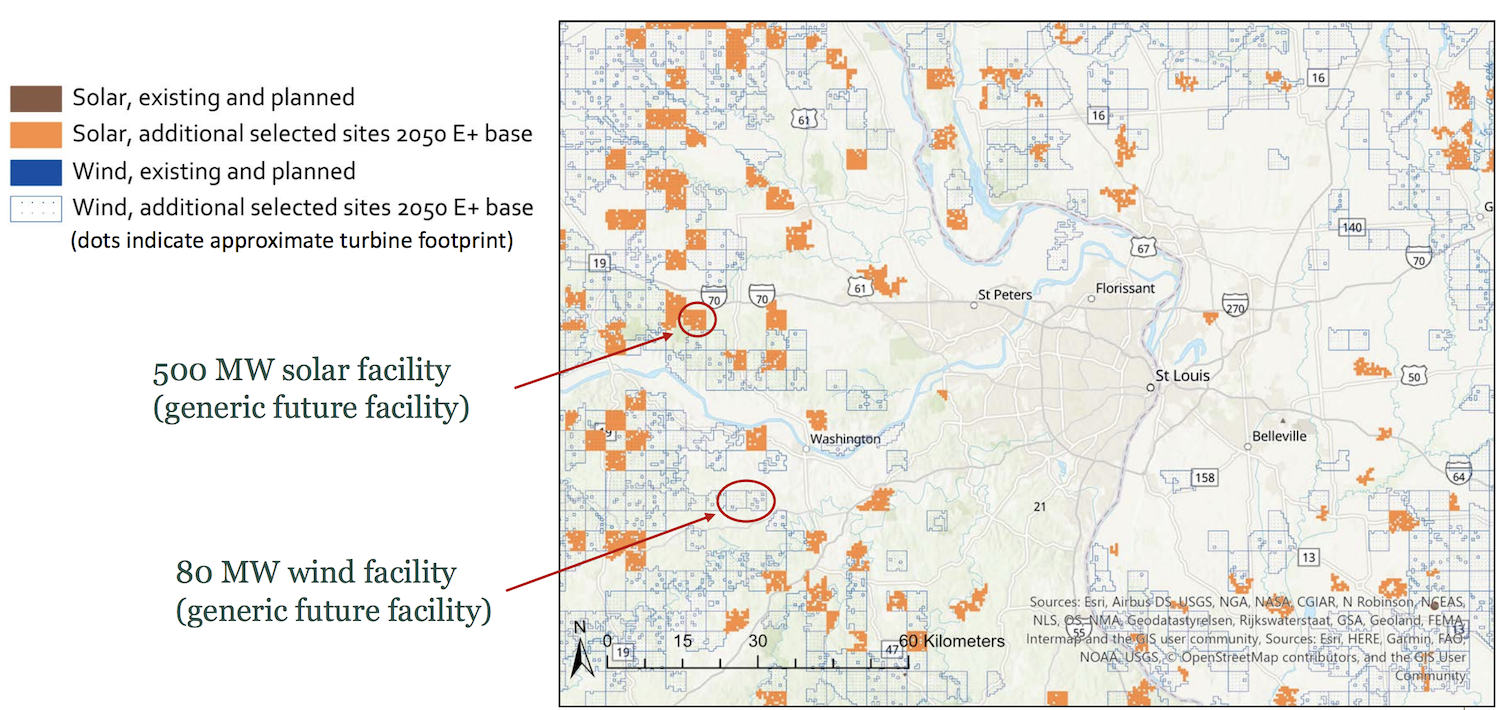
To achieve net-zero emissions, models have to phase out fossil fuels (at least without CCS) or include enough CO2 removal to balance out any residual positive emissions. However, not all fossil fuels are created equal, and all three models show a very rapid phase-out of coal and a much more gradual phase-out of natural gas. The figure below shows both coal use (solid lines) and the natural gas (dashed lines; excluding CCS) over time across the three models.
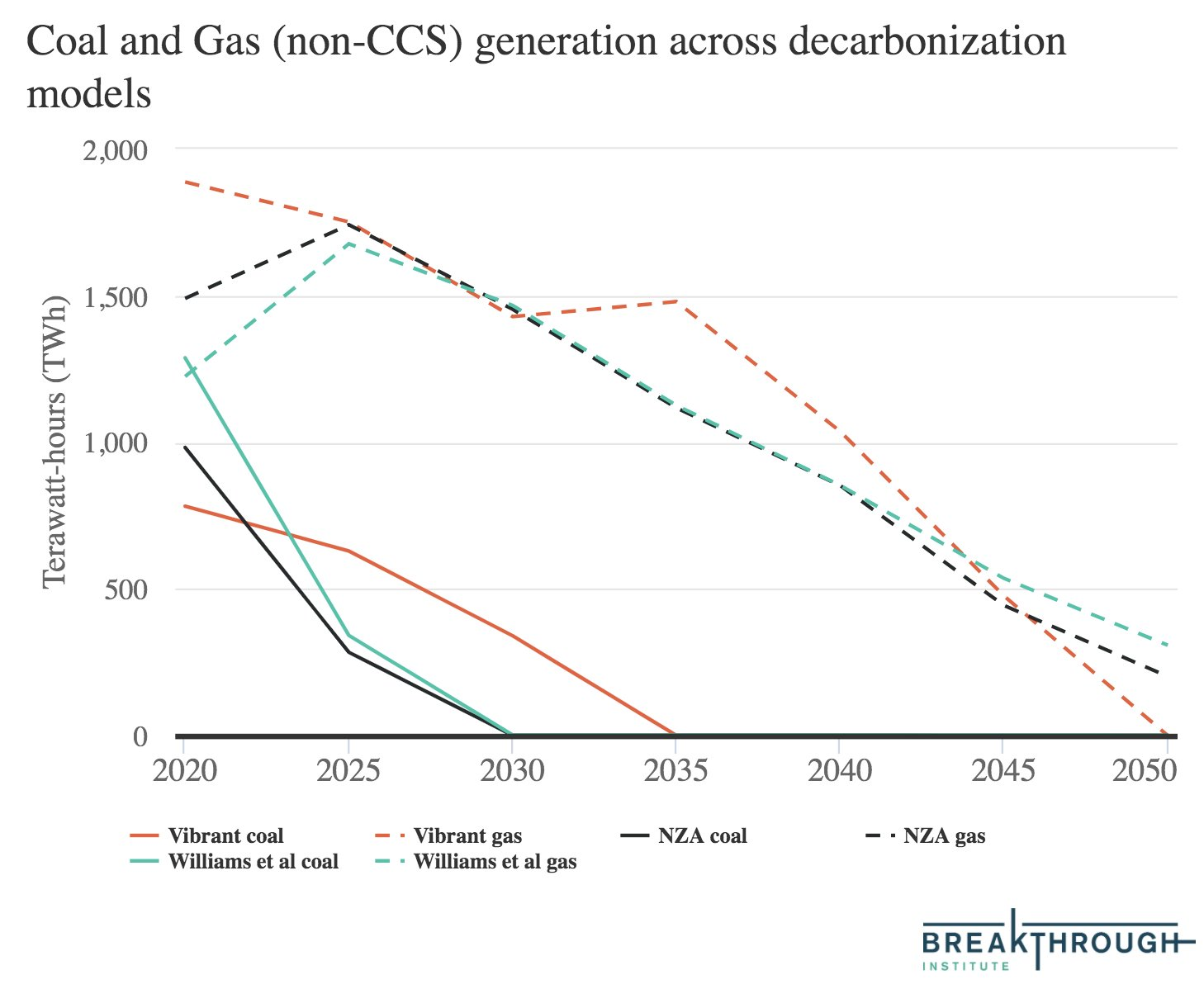
US coal generation falls dramatically from current levels, reaching zero before 2030 in the NZA and Williams et al. studies, and before 2035 in the Vibrant study. Natural gas generation, by contrast, stays close to today’s levels through 2030 and remains around 50% below current levels by 2040. It is only by 2050 that gas without CCS is eliminated in the Vibrant analysis and mostly gone in NZA and Williams et al.
Modest amounts of gas with CCS are used in both Vibrant and NZA, though it does not exceed 400 TWh at any point in any of the models. Vibrant is more bullish on gas with CCS in the short-term, showing substantial deployment by 2030 but falling after that as other cost-effective alternatives become available. NZA, by contrast, has relatively limited gas with CCS until after 2030, with continued growth up to 230 TWh by 2050.
While natural gas generation declines gradually over time in all three decarbonization models, the same is not true of gas capacity. Somewhat surprisingly, both NZA and Williams et al. actually have natural gas capacity at or above current levels through 2050. The figure below shows both natural gas capacity (solid lines; in gigawatts) and capacity factors (dashed lines) for the three models.
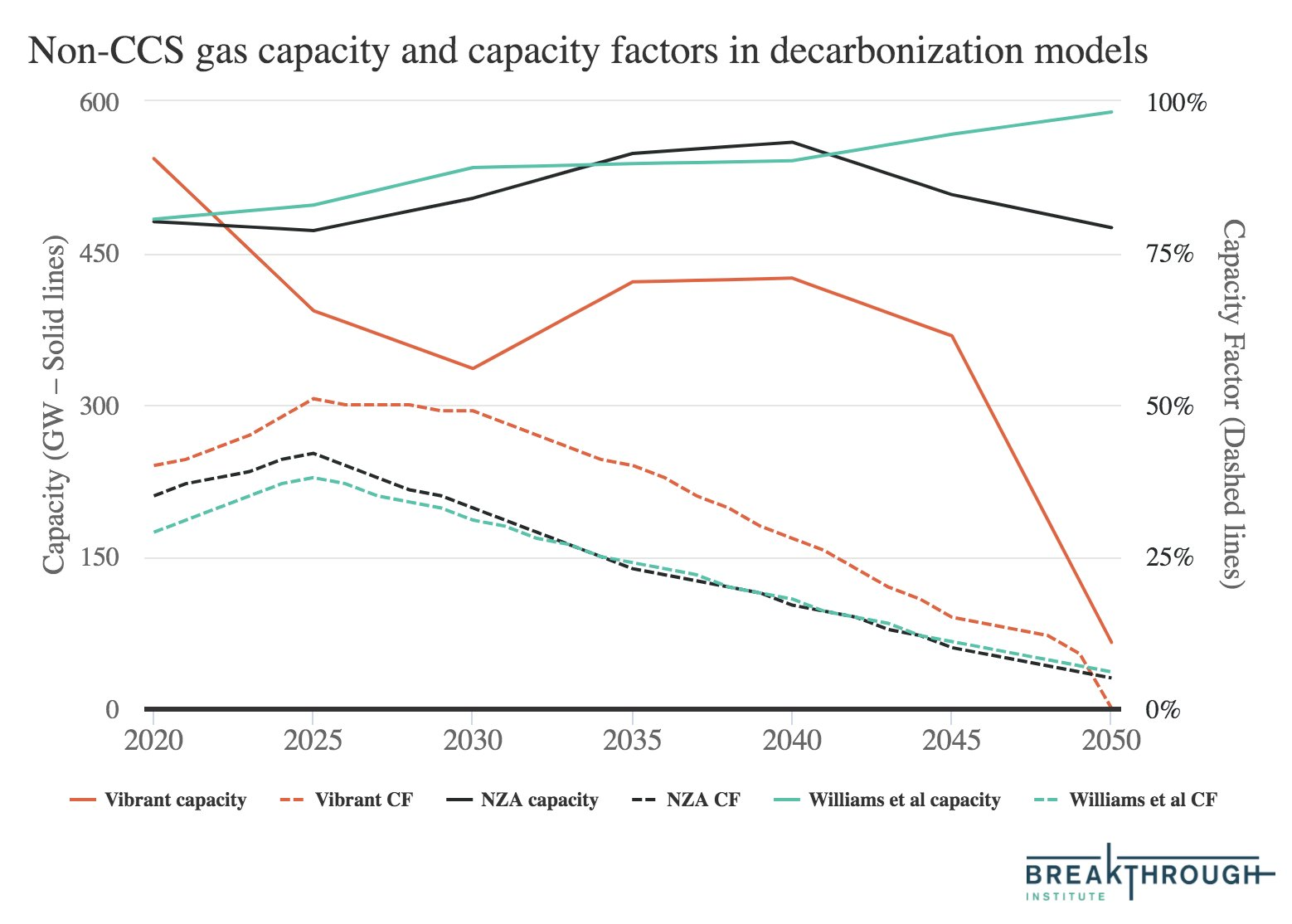
High natural gas capacity plays an important role in all three models to fill in the gaps in variable renewable generation as more wind and solar energy are installed on the grid. In the longer term, transmission expansion, storage, and development of alternative clean firm generation sources result in falling capacity factors, as gas increasingly operates as a peaking resource, reserved for either periods of exceptional demand or long periods of abnormally low variable renewable generation.
These decarbonization models suggest that while we need to move away from fossil fuels to decarbonize the economy, coal and gas play very different roles in the American energy system. Coal is a relatively inflexible resource that is poorly suited to a high-variable renewable generation system. Gas, on the other hand, has low capital costs and relatively high operating costs, making it easier to cost-effectively run with relatively low capacity factors and making it well-suited to support high levels of variable renewable deployments until alternatives are available.
Current technologies can get us a long way toward power sector decarbonization, but if we ever want to fully decarbonize — and move away from our reliance on natural gas — we need technologies such as grid-scale storage, advanced nuclear, gas with CCS, or hydrogen that are not mature today. We need to both accelerate the deployment of current cost-effective clean energy resources and invest considerably more in future technologies that will simultaneously lower system costs and enable deep decarbonization. As the NZA report argues, “the 2020s is the decade to invest in maturing and improving a range of technologies that improve options for the long term.”
Statements that “we have the technology we need and just need to build it” get it half right — we do need to build clean energy much more quickly than we are today. But we will need continued innovation in addition to this multi-decade buildout of clean energy. Clean energy policy is not zero-sum. Keeping this in mind as the US designs energy policy and wonks debate the merits of various decarbonization options will help ensure we pursue more cost-effective and socially-acceptable pathways going forward.
The future electricity mix is difficult to foresee perfectly, and history is a graveyard of failed energy model predictions. All models are wrong, as the saying goes, but some are useful. These deep decarbonization models give us a sense of what may be needed. We should not fixate too much on the specific generation mixes in any particular scenario, but we should take heed of where the models agree: on the importance of near-term renewables deployment, the medium-term role of gas capacity to fill in the gaps, and the importance of clean firm generation and complementary technologies to wean the power system off its dependence on natural gas in the longer term.