What Are the Land-Use Intensities of Different Energy Sources?
A new PLoS ONE paper models the land footprint of different energy system scenarios
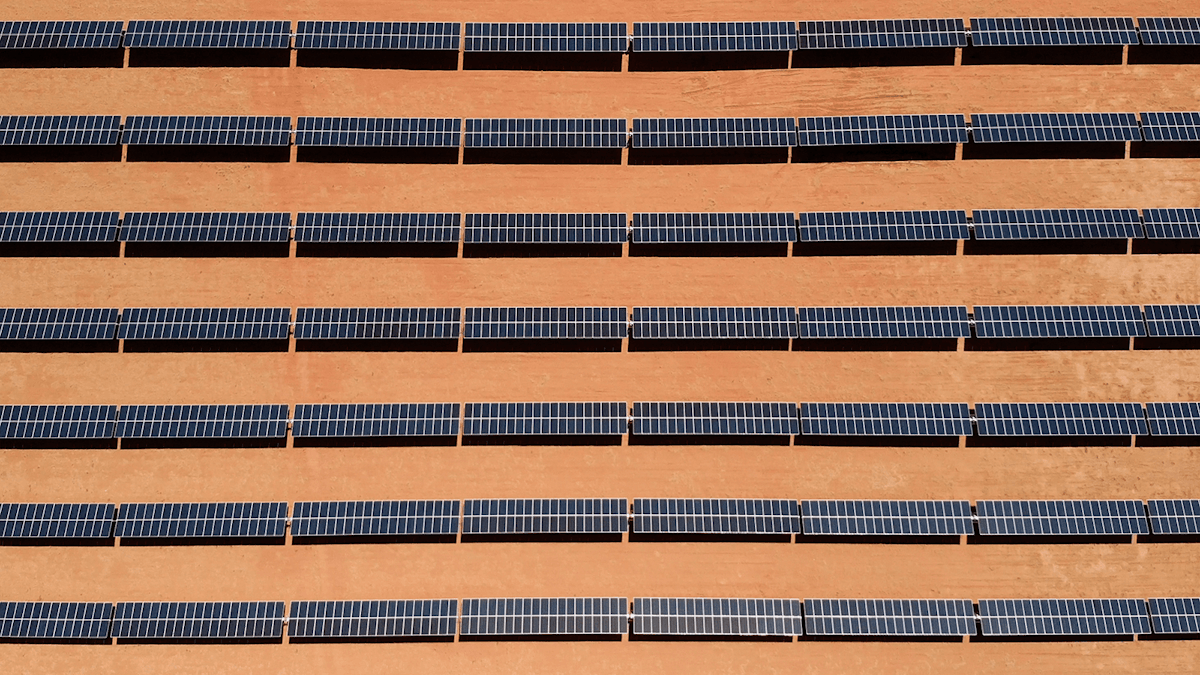
-
-
Share
-
Share via Twitter -
Share via Facebook -
Share via Email
-
Producing energy takes up land—a fact you could be forgiven for forgetting today. After all, the current energy system only occupies about 0.4% of ice-free land worldwide, most of it for hydroelectric power generation, a number dwarfed by agriculture, cities, and other anthropogenic land uses.
However, that balance is likely to change. In a new paper published in PLoS ONE, Jessica Lovering, Marian Swain, Rebecca Hernandez, and I study the land footprint of different energy sources, and our results are sobering. In a number of decarbonization scenarios, the land required for electricity generation expands by 30-80 million hectares. And that number grows to 80-800 million hectares if the spacing around the installations – for example, the area between individual wind turbines – is included. This expansion is on the same order as the projected growth in global urban areas (60-241 million hectares according to a study by Angel et al), and not far from the growth in cropland area that could result from feeding a growing population eating higher up on the food chain (160-320 million hectares across a range of studies).
Let's take a step back and look at how we arrived at these numbers. The object of our study was the land-use intensity of energy, defined as area per unit of energy production, or more specifically, the number of hectares per terawatt-hour of electricity generation in a given year.
We calculated land-use intensity not through modeled or theoretical values for area and electricity generation, nor by just looking at a small number of facilities and extrapolating, but instead by using real-world data for a large number of energy facilities. In total, we have 1,400 observations covering nine electricity sources—coal, natural gas, nuclear, wind, solar PV, concentrated solar, geothermal, hydroelectric, and biomass—across 73 countries and 45 US states. For some of these, we collected data from published studies, and for some we matched our own measures of area, assessed from Google Earth, to publicly available information on electricity generation. We include both the space needed for the power plants themselves (direct land use), and the space needed for upstream production of the fuels (indirect land use).
We also make a distinction between what we call "footprint" and "spacing" for natural gas and wind. Footprint area represents land directly covered by infrastructure, while spacing area is the entire area within the perimeter of a production site. For wind, for example, the footprint is just the area of the turbine pads and access roads, whereas the spacing is the area of the whole wind farm.
The results in Figure 1 show that the land-use intensities of the different electricity sources differ by four orders of magnitude — that's a factor of 10,000. (Note that the y axis is on a log scale, so the real differences are much larger than they appear.) Nuclear had the lowest median land-use intensity at 7.1 ha/TWh/year, and biomass the highest at 58,000 ha/TWh/year.
Other renewable electricity sources, as well as fossil fuels, fall between nuclear and biomass. The median footprint of wind, for example, is 130 ha/TWh/year; if spacing is included, it is 12,000. The corresponding numbers are 2,000 for ground-mounted solar PV, and fossil fuels range from 410 (the footprint of natural gas) to 1,000 for coal, with natural gas spacing reaching 1,900.
Two sources with particularly low footprints are rooftop solar, which we count as having a footprint close to zero (the area required for producing materials is negligible), and residue biomass, with a land-use intensity of 130 ha/TWh/year. However, there are serious technical and economic limitations to how much these two sources can scale up.
To get an even better sense of how energy sources perform, we compared the land footprint to the greenhouse gas footprint. Ideally, we're looking for electricity sources with low footprints for both — that is, in the bottom left corner of figure 2 below. Nuclear looks good, as do wind (footprint) and rooftop ("integrated") solar. Hydroelectric, solar CSP, ground-mounted solar PV, and wind (spacing) have relatively low carbon footprints, but higher land-use intensity. Fossil fuels have land-use intensities that are comparable (in terms of order of magnitude) to hydroelectric, solar CSP, and ground-mounted solar PV, but have high carbon emissions, so they are no environmental winners either.
A tricky question when it comes to wind is whether to look at the footprint or the spacing. It really depends — the entire spacing area can matter in terms of just the sheer feasibility of scaling up, as well as for habitat fragmentation and other environmental impacts. Yet land in between wind turbines can sometimes be used for other purposes, in which case the footprint might be the relevant metric.
Knowing these land-use intensities helps us understand the implications of a future energy system that is bound to look very different from today—both as a result of technological advances and, hopefully, strong action to mitigate climate change. We don't know what shape that energy system will take, but we can look to different visions for it that have been presented. To do so, we chose ten prominent scenarios, ranging from the International Energy Agency's business-as-usual scenario to ambitious decarbonization pathways from Greenpeace, World Wildlife Fund, Mark Jacobsen and Mark Delucci, and others. We calculated the total land footprint of these scenarios by applying our estimated land-use intensities to the amount of electricity coming from each source in these scenarios' energy mixes.
As noted at the beginning, virtually all the scenarios do involve at least a doubling of the land footprint of energy, with the scenarios from Jacobson and Delucci having by far the largest land footprint, on the order of 500-900 million hectares, by 2030. The latter figure is roughly the same as the total land area of the United States!
The actual environmental impacts of this land use depend on a variety of factors, such as whether ground-mounted solar PV can be sited on already disturbed land, the scalability of rooftop solar, and whether wind can be co-sited with agriculture. But we are already seeing new energy facilities competing with other land uses. Today, there is twice as much ground-mounted as rooftop solar, and this is, in places like California, often located in natural habitats like scrublands and shrublands. Only half of US wind is currently co-sited with agriculture.
Even if there are some ways of mitigating the environmental impacts of using land for energy production, in other words, the energy system of the future might have some very serious environmental consequences—especially habitat loss and associated biodiversity loss—as a result of its land footprint. If you're not already paying attention to the land-use intensity of energy, now is a good time to start.