Advanced Nuclear Moves Beyond Water and Into Salt
As engineers look at the challenge of decarbonizing the electricity system and everything else, another reactor type stands out as a potentially valuable tool: molten salt.
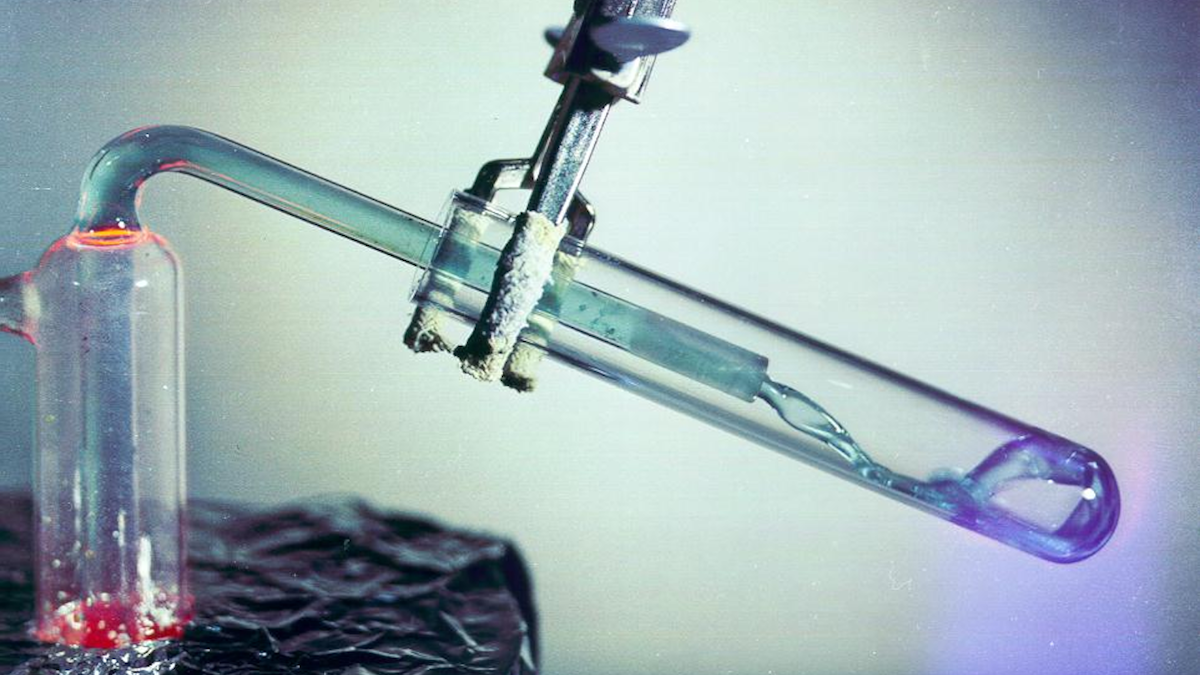
-
-
Share
-
Share via Twitter -
Share via Facebook -
Share via Email
-
As engineers look at the challenge of decarbonizing the electricity system and everything else, another reactor type stands out as a potentially valuable tool: molten salt.
There are a variety of different molten salt designs, but these reactors, known as MSRs, all have two things in common. First, the salt works well at very low pressure, or no pressure at all, which simplifies the construction of the reactor and reduces the possibility of leaks. (And if molten salt leaks, it just solidifies, like candle wax.) Second, the salt readily absorbs large quantities of heat, making it a very good heat transfer agent that allows the reactor to operate at a very wide range of temperatures. (And a core meltdown is out of the question; it’s already melted.)
In contrast to MSRs, the current generation of reactors use water in the place of salt, and the water is kept at very high pressures—up to 2,200 pounds per square inch in the design that is used most frequently. Pressure is needed to limit boiling or to prevent it completely. Pressures that high require thick reactor vessels and piping. They also require preparation against leaks that would have tremendous energy behind them.
Because an MSR can achieve higher temperatures safely, it yields greater efficiency in electricity production; that is, it makes more kilowatt-hours per unit of heat produced by the reactor. And because heat from a molten salt reactor is at a higher temperature, it can do more kinds of work, including powering a variety of industrial processes.
Salt has another fundamental difference from water. In a reactor, water slows down the neutrons, the sub-atomic particles that sustain the chain reaction, to a speed that is more likely to result in the neutrons splitting another atom. In that job, water is called a moderator.
Salt does not slow down the neutrons. Some designs add graphite as a moderator, but others do not; they use the neutrons at the high speed and high energy at which they are emitted from the nucleus as it splits. These “fast” neutrons can split atoms of uranium and other materials that ordinarily are not split in today’s reactors. That leads to a much more thorough use of nuclear fuel. In some reactor configurations, fast neutrons can also split atoms that are produced through fissioning uranium, and that are today considered waste. In an MSR, those atoms can give more energy, and be converted into other materials that are easier to dispose of.
In addition to consuming an extremely high proportion of their fuel, MSRs don’t have to stop for refueling. As the reactor runs, a small stream of the fuel can be removed, and fission products—the fragments of atoms created when atoms are split—can be chemically separated, much as dialysis cleans the blood. With no fission products to get in the way, the chain reaction can continue, as new fuel is pumped in.
And while LWRs are usually inflexible in their production, molten salt can be ramped up and ramped down quickly, making MSRs a good dance partner for variable wind and solar production.
Molten salt reactors have some built-in safety features as well. Some use fuel dissolved in the salt, and as the reactor gets hotter, the salt expands, and thus the fuel is diluted, making the conditions for fission less favorable. That tends to make the reactor temperature self-limiting.
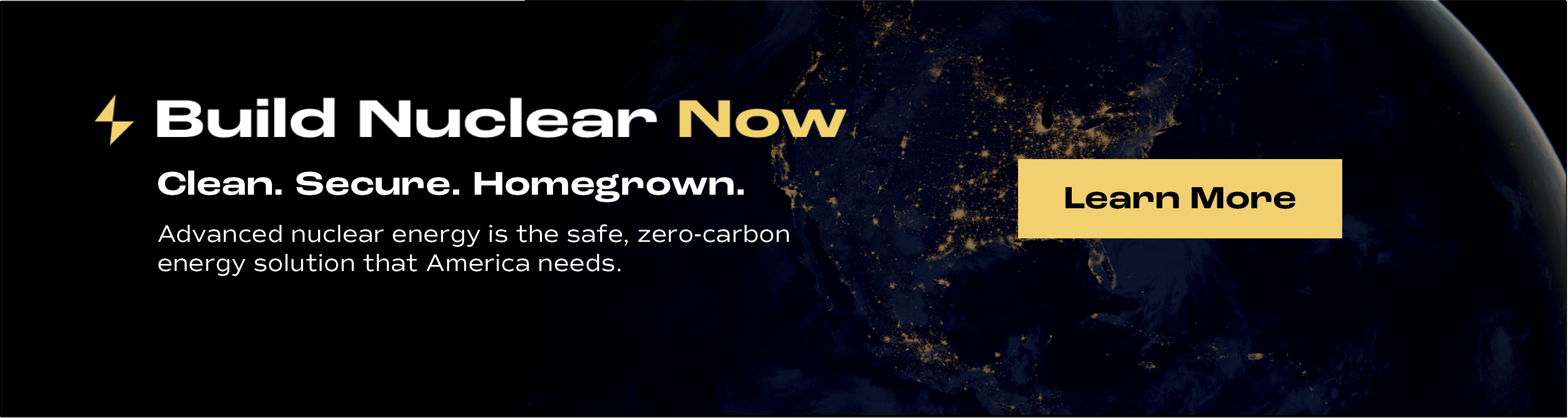
What’s the status of salt reactors now?
The Energy Department’s Advanced Reactor Demonstration Program is advancing three kinds of molten salt reactors. One, called Natrium, is a joint venture between GE-Hitachi and TerraPower, the company founded by Bill Gates. (Natrium is the Latin name for sodium.) It uses sodium in the reactor, and salt in a giant tank, to store the heat.
The heat can be used to boil water, and the steam spins a turbine and makes electricity. That can be done as the heat is produced by the reactor, or a few hours later; the idea is to build up the heat in the storage tank during daylight hours, when a lot of solar panels are productive, and make the electricity around sunset when demand is high but solar won’t work.
Natrium is a fast reactor. A second project funded by the Advanced Reactor Demonstration Program, called Kairos, uses graphite to “moderate” the speed of the neutrons, just as today’s reactors use water as a moderator. Kairos uses “pebble” fuel, which can tolerate high heat. The wide range of operating temperatures of the salt provides a huge safety margin, and the reactor will produce very high-temperature heat at low pressures.
A third project funded by the Advanced Reactor Demonstration Program is the Molten Chloride Fast Reactor, which uses utilizes liquid salt as both fuel and coolant in the reactor core. As the salt passes through the core, enough neutrons are reflected back to the fuel in the salt for sustainable fission to take place.
A fourth salt design, a step further back in the path to commercialization, loads the salt with thorium, an abundant element whose atoms are not easy to split. But in a reactor, thorium atoms will absorb neutrons emitted by fission, and turn into uranium atoms, which can be split. The company is called Flibe Energy, for the mixture of salts it uses, lithium fluoride and beryllium fluoride (FLiBE).