Fixing Nitrogen
The Hidden Footprint of Making All Farms Organic
-
-
Share
-
Share via Twitter -
Share via Facebook -
Share via Email
-
Organic farming, which prohibits the use of synthetic fertilizers, is often touted as the solution to many of agriculture’s environmental impacts. For instance, Sweden’s Environmental Party calls for 100% organic food production in the European Union.1
There are well-worn arguments about whether this would be good for the environment or even possible. Environmental and agricultural scientists note that organic farms typically have 20% lower yields than conventional farms, requiring more land to produce a given amount of food.2,3 This means less land for wildlife habitats or other purposes. Organic farmers and advocates argue that this yield gap can be closed, perhaps with more research or support for organic farming.4
Yet there’s a crucial difference between organic and conventional farming that’s missing from the discourse. Without synthetic fertilizers, farms need to grow additional crops — legumes — to provide nitrogen, and these require extra land. This "shadow land footprint" of organic farming and other agricultural systems that don’t use synthetic fertilizer is equally, if not more, important than the yield gap. Taking it into consideration highlights how continuing to use synthetic nitrogen, albeit more efficiently and wisely, is key to growing food with minimal environmental impact.
Providing enough nitrogen to crops has been the central challenge of agriculture
The element nitrogen is essential to crop production.5 It is present in every living cell and responsible for the growth of plants, particularly the cereal grains we consider staples. Although nitrogen makes up almost 80% of Earth’s atmosphere, plants cannot use it in this form. The forms of nitrogen that they require for growth — mostly ammonia and nitrate — are scarce. In fact, a shortage of this usable (or reactive) nitrogen is often the factor that most limits crop production.6
Societies have perennially sought to overcome this challenge through two basic approaches: fixing nitrogen and recycling it. Reactive nitrogen can be added by fixing (transforming) atmospheric nitrogen. Leguminous plants such as soybeans, lupine, and clover can do this, as can humans through industrial processes we have developed. Nitrogen can also be recycled, using nutrients in animal manure, spoiled food, crop residues, and other so-called waste products to return nitrogen to crops. However, a portion of recycled nitrogen is inevitably lost and so there is a constant need to add fixed nitrogen.
Historically, farmers relied upon techniques such as slash-and-burn and fallowing land for years to provide enough nutrients to crops. Families would go through great lengths to cut and burn trees and other biomass, clearing the land, and releasing the nitrogen stored in the plants to the soil. After farming the land until they drained the soil of nutrients, they would allow it to lie fallow, letting trees and other plants grow and rebuild the nutrient supply before repeating the process. Each plot of land generally grew trees and weeds for more time than it grew crops. This limited the total amount of food a village or society could grow.7
The low productivity of land under slash-and-burn forced people to find new methods of fertilizing crops. At least eight thousand years ago, farmers in the Middle East added nitrogen to their soil by planting legume crops.8 Likewise, the Romans recognized the value of legumes. The statesman Cato wrote that “crops which fertilize land [are] lupines, beans, and vetch,” and the agricultural writer Columella wrote that planting and ploughing in lupine “will have the effect of the best manure.”9 The added nitrogen from these legume crop rotations enabled farmers to increase yields and fallow their land less.10
As populations grew, farmers found new methods of providing nitrogen. Farmers in China found ways to recycle 70-80% of their animal and human waste, carting “night soil” (a euphemism for excrement) from cities to the countryside where it was composted and applied to crops.11 Similarly, farmers in Europe developed increasingly complex crop rotations that included legumes. During the 17th and 18th centuries, British farmers began rotating cereal crops, such as wheat and barley, with crops that livestock could graze on, including turnips and clover. The large amounts of nitrogen added from clover and manure led to a dramatic increase in productivity often credited with jumpstarting England’s industrial revolution.12,13
Despite these innovative and laborious methods of fixing nitrogen through legumes and recycling nutrients in waste, the need for nitrogen only grew. During the 19th century, the populations of the United States and Europe were growing at an unprecedented pace — the U.S. population increased tenfold and Britain’s more than tripled.14 To raise farm productivity, these imperial powers started to import nitrogen-rich guano (bird or bat excrement) from Caribbean and Pacific Islands, one of which had deposits up to 200 feet deep.15 For the same reasons, they mined sodium nitrate from the deserts of Chile.16
However, these sources were finite and population growth appeared to outpace nitrogen availability. At the turn of the century, there were explicit warnings that the world was running out of nitrogen. Sir William Crookes, president of the British Association for the Advancement of Science, warned of famine if new sources of nitrogen were not discovered or synthesized. He declared that "all civilized nations stand in deadly peril of not having enough to eat... We are drawing on the earth's capital, and our drafts will not perpetually be honored."17
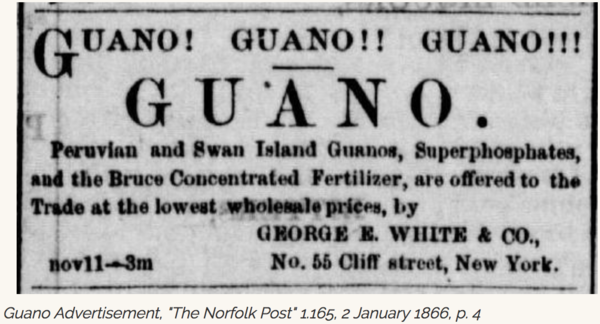
Source: Totten, Kimberly (2016). Smithsonian National Museum of History Blog.
Synthetic fertilizer changed everything
In the early 1900s, German scientists developed the Haber-Bosch process for producing fertilizer at an industrial scale. The energy-intensive process converts atmospheric nitrogen to ammonia, a form that plants can easily use, using a chemical reaction with hydrogen under high temperatures and pressures. This enabled the production of plentiful ammonia fertilizer, freeing farmers from their dependence on far-flung reserves of guano and other nitrogen sources.
It also dramatically raised crop production. A group of prominent nitrogen researchers recently wrote in the science journal Nature that nitrogen fertilizer synthesized through the Haber-Bosch process increased the number of people an average acre of land could feed up to 60%.18 They, and others, credit it with feeding billions of people — about half the current world population.19
Synthetic nitrogen from the Haber-Bosch process enabled this historic increase in productivity by making nitrogen more easily available. Farmers did not have to limit their crop production to what manure, compost, and other recycled nutrients could sustain. Additionally, synthetic fertilizer provided nitrogen to crops in a chemical form that they could more easily absorb.
The shadow footprint of nitrogen fixation
The yield increases and benefits of synthetic fertilizer persist today and remain essential. Many studies have found that even the best-managed organic farms, which do not use synthetic fertilizer, have 10-25% lower yields on average than similar farms that do.20,21 The difference in yields is even greater for some of the most commonly grown crops, such as wheat.22 Differences in pest management play a role in this, but the primary cause is likely that organic farms are more nitrogen-limited; it is more difficult to provide plants with the optimal amount of nitrogen when and where they need it.23 These lower yields mean that it takes more land to grow the same amount of food with organic fertilizers, such as manure, as it does with synthetic ones.
What is too often overlooked is that additional land is also needed to produce or fix nitrogen when synthetic fertilizers are not used. This has been called the shadow land footprint.24 Just as land was historically set aside to regenerate soil nutrients, so too must it be today to provide nitrogen for farmers who don’t use synthetic fertilizer.
Farmers must set aside land to plant nitrogen-fixing cover crops instead of crops for harvest. To provide the most nitrogen, these are plowed back into the soil. This has a tremendous impact on land use, particularly when considered globally. Traditionally, farmers have reserved on the order of 25-50% of their farmland for nitrogen-fixing legumes, either to be grazed on by cattle or incorporated into the soil.25 The exact amount depended on the legume, the harvested crop, and the availability of other nitrogen sources, such as manure, among other factors.
Regardless, these nitrogen-fixing crops — though beneficial in many ways — can displace edible crops, cutting the amount of food that can be produced on a plot of land, and thereby requiring more land to feed people. Synthetic fertilizer allowed farmers to grow crops continuously, planting and harvesting crops every year rather than setting it aside for N-fixing crops. This has shrunk the shadow land footprint of agriculture — by as much as 50% for some farms — helping the world use less land for agriculture per person than it has since the Bronze Age.26
The shadow footprint of recycling nitrogen
Unlike planting legumes, applying recycled nitrogen such as manure and compost doesn’t require additional land use. They are byproducts of existing processes and generally would be produced regardless of whether farmers applied them as fertilizer.27
However, these nitrogen sources are derived from plants, many of which are fertilized with synthetic fertilizer. Take manure, for example. The nitrogen in manure is obtained from livestock eating pasture, forage, or feed crops. These plants take up nitrogen by fixing it themselves, from nitrogen in the soil fixed by other legumes, or from applied synthetic nitrogen.
Farmers, whether organic or conventional, get much of their manure from livestock that ate conventionally grown feed and forages that were fertilized with synthetic nitrogen. One survey of organic farms in France found that synthetic nitrogen from conventional agriculture accounted for more than 80% of nitrogen in the manure they used.28 If all farming was organic and synthetic fertilizer was phased out entirely, this manure nitrogen would need to be added to the system by legumes, further increasing the food system’s shadow land footprint.
Thought experiment: the land-use cost of replacing synthetic fertilizer with nitrogen fixation only
Organic farming and other systems that do not use synthetic fertilizer comprise a minority of production today, but are growing more widespread. Although synthetic fertilizer has been key to feeding a growing population, it also contributes to some of today’s most daunting environmental challenges.29 There’s wide agreement that we should reduce various forms of nitrogen pollution. Many believe that avoiding synthetic fertilizer is the best way to get the job done. Yet this would backfire, dramatically increasing agriculture’s land and environmental footprint.
Let’s consider a thought experiment: what would happen if we tried to replace current global synthetic fertilizer with nitrogen from legumes and manure while still meeting today’s food demand? You could also envision this happening on a smaller scale, such as in a single country.
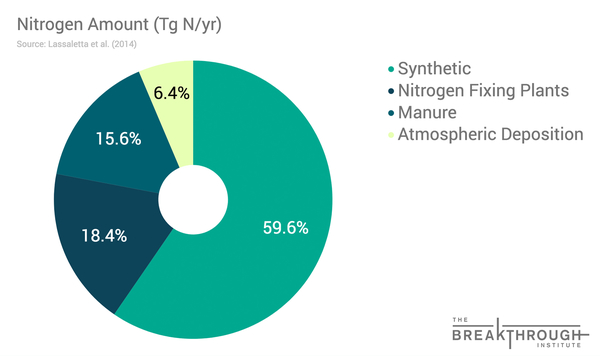
Figure 1
Since synthetic fertilizer provides nearly 60% of current nitrogen for producing crops, eliminating it without making any other changes would require far more farmland to fix enough nitrogen to maintain production (Figure 1).30,31 The world would need to more than double the amount of cropland, increasing it by an area larger than all of Russia, to produce as much food as it does today.32,33
Yet farmers could replace some of the nitrogen from synthetic fertilizer by planting legumes or by recycling nitrogen in manure and other waste products. They could also replace existing cover crops with ones that fix more nitrogen. Clover, for example, is one of the highest-fixing cover crops; it fixes twice as much nitrogen as vetch and far more than most other crops.34 We estimate that swapping out existing leguminous cover crops exclusively with clover would replace less than 3% of the nitrogen provided by synthetic fertilizer today.35 Increasing nitrogen fixation from legumes grown for harvest, for example by switching from chickpeas to soybeans, could provide a similar amount.36
Farmers that don’t currently plant cover crops could also start. In the United States, only 2% of cropland is cover cropped.37 There appears to be a large opportunity to fix more nitrogen on the other 98% of farmland. However, both in the US and globally, many farmers are already planting crops for harvest on this land during the winter, when cover crops could otherwise be planted. One study estimated that half of global cropland is already planted in winter crops and 25% is unsuitable for various reasons, such as low water availability. Therefore, no more than 25% of the world’s cropland area could potentially be cover cropped.38
Planting cover crops on this land would help replace nitrogen from synthetic fertilizers, but would have its own shadow land footprint. If farmers chose (and were able) to plant crops for harvest, instead of cover crops, they could help reduce agriculture’s overall land use. For instance, if 20 million acres of cropland added an additional harvest of cereal crops, doubling their yields, that could reduce the amount of farmland needed elsewhere to feed people by up to 20 million acres. A similar dynamic has been observed globally, with yield increases reducing the rate of cropland expansion by about 1.5% per year.39 Therefore, increasing plantings of cover crops when crops for harvest can be planted and yields increased can require more global agricultural land use.
Still, a significant amount of nitrogen could be fixed without this shadow footprint by planting cover crops during times of the year when other crops can’t be grown. A 2015 study from the International Food Policy Research Institute estimated that at least 13% of global cropland could add another cereal crop.40 This suggests that almost half of the area that could support a cover crop could not support a cereal crop. If this land — no more than 12% of global cropland — added high nitrogen-fixing cover crops, the fixed nitrogen could replace up to 38% of current synthetic fertilizer use.41
This is an upper bound or maximum estimate – fixing this level of nitrogen is not necessarily feasible. Planting legumes that fix more nitrogen is not a simple change for everyone; there are reasons why all farmers do not already grow these crops. For example, common cover crops are not suited to every climate, seeds and irrigation are not always available, and some crops must remain in the ground too long to be economical. Even if we assume this level of fixation is possible, it still cannot replace the majority of nitrogen from synthetic fertilizer.
What about both nitrogen fixation and recycling — could manure and compost fill the gap?
What about manure and compost? Could they replace synthetic nitrogen without requiring greater land use? Not even close.
It’s certainly true that we could more efficiently use animal manure, food waste, and sewage by turning more of it into plant fertilizer. Most nutrients in manure are currently not used by plants. Instead, they’re lost as gaseous emissions or leach into bodies of water.42 We should certainly use manure and other nitrogen sources as efficiently as possible when cost-effective.
Yet using the best manure management and application practices could only reduce nitrogen losses by 10–20 million tonnes globally.43,44 This wouldn’t be close enough to displace synthetic fertilizer. At most, it could replace about 21% of current fertilizer use.45
Similarly, applying half of all nutrients in food waste and sewage to cropland — more than 10 times the amount currently applied — could replace no more than 16% of current synthetic nitrogen.46
In any scenario, eliminating synthetic fertilizer would require massive farmland expansion
Even with the most optimistic scenarios for improving nitrogen fixation and nutrient recycling, farmland would need to dramatically expand to entirely replace synthetic fertilizer. These improvements could provide no more than 90% of the nitrogen currently provided to crops.47 Assuming a proportionate drop in production, at least 12% more cropland (nearly 500 million acres) would be needed to maintain production.48
Additionally, due to organic farming’s yield gap, an additional 25-33% more land would be needed.49 But to make up for both the yield gap and the shadow footprint, we would need at least an extra Australia-sized amount of land — between 40% and 100% more agricultural land than the amount we use today.50
The full environmental costs of such an expansion are difficult to fathom. Forests would be logged. Habitats lost. Carbon released from trees and soil. We can certainly quibble about the exact size of the shadow footprint and how to calculate it. Perhaps the yield gap is closer to 15%, perhaps 25%. Maybe 15% of land is suitable for fertilizer crops, maybe 5%. But the fundamental conclusion remains: a fertilizer-free food system would mean converting an enormous amount of land to agriculture — at least 1 billion acres.
And this is a lower bound estimate. Using mid-range assumptions, it appears more likely that closer to 3 or 4 billion acres of additional cropland would be needed to meet today’s food demand without synthetic fertilizers.51 Given the many economic, technical, and biological barriers, the amount of non-synthetic nitrogen the world could realistically generate is likely far less.
Even modest increases in organic farming would mean land expansion
Clearly, eliminating synthetic fertilizer is a far-fetched idea. But what about scaling up organic farming just a little?
Elimination of synthetic fertilizer on any farmland requires more land, as the yield gap between conventional and organic farms suggests. But the scale of the shadow land footprint depends on farmers’ ability to fertilize crops with excess manure and to increase fixation from legumes. If farmers maintain existing fixation rates and rely only on manure, they will quickly hit a limit where there is no more manure nearby to purchase. In the United States, for example, most regions already do not have enough manure to provide even half of the nitrogen their crops need.52,53
Even if farmers can increase fixation by planting more legumes, a small increase in organic farming globally will require more farmland. Research led by the Research Institute of Organic Agriculture estimated that converting just 20% of farmland to organic production would require 5% more land, and their results underestimated the actual land needed, as Dan Rejto has about written elsewhere.54
Synthetic fertilizer isn’t going anywhere, and that’s a good thing
Synthetic fertilizers have clearly enabled the world to produce more food with less land than would have otherwise been possible. We should recognize that sustainable agriculture requires continued and more efficient use of synthetic fertilizer. This includes reducing the use of synthetic fertilizer where it is overapplied, such as through the use of precision farming technologies. But it also includes using fertilizer to boost yields where they are low and enable people to feed themselves while using less land. Considering synthetic nitrogen’s role in reducing land use and its importance in producing manure, sustainable and organic agriculture advocates should reconsider its merits.
References
1. https://www.mp.se/politik/mat-och-jordbruk
2. Ponisio, L. C., M’Gonigle, L. K., Mace, K. C., Palomino, J., de Valpine, P., & Kremen, C. (2014). Diversification practices reduce organic to conventional yield gap. Proceedings of the Royal Society of London B: Biological Sciences, 282(1799). Abstract. Retrieved from http://rspb.royalsocietypublishing.org/content/282/1799/20141396
3. This is an average of the gap observed in many studies of different crops in different environments. The gap varies by crop as well as location, management practices and many other factors.
4. See, for example, Ponisio et al. (2014). p. 5
5. For simplicity and ease of reading, We refer to chemical compounds containing nitrogen, such as ammonium and nitrate, as nitrogen, except when discussing forms of nitrogen pollution.
6. Smil, V. (2001). Enriching the earth : Fritz Haber, Carl Bosch, and the transformation of world food production. MIT Press. p.xiii
7. Smil, V. (2001) p.22
8. Smil, V. (2001) p.29
9. Savio, Hannah L. (2011) "Sustainable Agriculture in Ancient Rome." Senior Capstone Projects. http://digitalwindow.vassar.edu/senior_capstone/2 p.31-32
10. Smil, V. (2001) p.32-33
11. Smil, V. (2001) p.27
12. Bruns, H. A. (2012). Concepts in crop rotations. In Agricultural Science. InTech. http://cdn.intechopen.com/pdfs/36492/InTech-Concepts_in_crop_rotations.pdf p. 25
13. Smil, V. (2001) p.32-33
14. Max Roser and Esteban Ortiz-Ospina (2017)—‘World Population Growth.’ Published online at OurWorldInData.org. Retrieved from: https://ourworldindata.org/world-population-growth/
15. Totten, Kimberly. (2016). “What a load of guano: 5 facts you didn't know about bird poop.” Smithsonian National Museum of History Blog. http://americanhistory.si.edu/blog/what-load-guano-5-facts-you-didnt-know-about-bird-poop
16. Smil, V. (2001). p.46-48
17. Smil, V. (2001). p.58
18. Erisman, J. W., Sutton, M. A., Galloway, J., Klimont, Z., & Winiwarter, W. (2008). How a century of ammonia synthesis changed the world. Nature Geoscience, 1(10), 636–639. https://doi.org/10.1038/ngeo325 p. 637.
Calculated: The article states the number of number of humans supported per hectare of arable land has increased from 1.9 to 4.3 persons between 1908 and 2008 (126%) and that 30-50% of this yield increase was due to mineral fertilizer application. 30 to 50% of the yield increase is 38 to 63%.
19. Erisman et al. (2008). p. 637
20. Ponisio, L. C., M’Gonigle, L. K., Mace, K. C., Palomino, J., de Valpine, P., & Kremen, C. (2014). Diversification practices reduce organic to conventional yield gap. Proceedings of the Royal Society B: Biological Sciences, 282(1799), 20141396–20141396. https://doi.org/10.1098/rspb.2014.1396. p. 4
21. Seufert, V., Ramankutty, N., & Foley, J. A. (2012). Comparing the yields of organic and conventional agriculture. Nature, 485(7397), 229–232 https://doi.org/10.1038/nature11069 p. 230.
22. Ponisio et al. (2014). p. 45
23. Berry, P. et al. Is the productivity of organic farms restricted by the supply of available nitrogen? Soil Use Manage. 18, 248–255 (2002) in Seufert, V., Ramankutty, N., & Foley, J. A. (2012). Comparing the yields of organic and conventional agriculture. Nature, 485(7397), 229–232 https://doi.org/10.1038/nature11069 p. 230.
24. https://thebreakthrough.org/archive/how_landefficient_is_organic_a
25. Smil, V. (2001) and Crews, T.E., (1993). Phosphorus regulation of nitrogen fixation in a traditional Mexican agroecosystem. Biogeochemistry 21, 141–166 in Crews, T. E., & Peoples, M. B. (2004). Legume versus fertilizer sources of nitrogen: ecological tradeoffs and human needs. Agriculture, Ecosystems & Environment, 102(3), 279–297. https://doi.org/10.1016/j.agee.2003.09.018 p. 280
26. Klein Goldewijk, K., A. Beusen, J.Doelman and E. Stehfest, New anthropogenic land use estimates for the Holocene; HYDE 3.2. In Max Roser and Hannah Ritchie (2017)—‘Yields and Land Use in Agriculture’. Published online at OurWorldInData.org. Retrieved from: https://ourworldindata.org/yields-and-land-use-in-agriculture/
27. Although more research is warranted to examine whether demand for manure and revenue from selling manure leads to increases in animal production.
28. Nowak, B., Nesme, T., David, C., & Pellerin, S. (2013). To what extent does organic farming rely on nutrient inflows from conventional farming? Environmental Research Letters, 8(4), 44045. https://doi.org/10.1088/1748-9326/8/4/044045 p. 3
29. e.g. Robertson, G. P., Vitousek, P. M., & Kellogg, W. K. (2009). Nitrogen in Agriculture: Balancing the Cost of an Essential Resource. Annual Review of Environment and Resources, 34(1), 97–125. https://doi.org/10.1146/annurev.environ.032108.105046
30. Lassaletta, L., Billen, G., Garnier, J., Bouwman, L., Velazquez, E., Mueller, N. D., & Gerber, J. S. (2016). Nitrogen use in the global food system: past trends and future trajectories of agronomic performance, pollution, trade, and dietary demand. Environmental Research Letters, 11(9), 95007. https://doi.org/10.1088/1748-9326/11/9/095007 Supplementary Data 7.
31. There would also be indirect reductions since some synthetic fertilizer usually escapes to the atmosphere upon application (through volatilization) and then falls back onto cropland through a process known as atmospheric deposition. However, this deposition provides a small portion of the nitrogen crops use so eliminating it would likely not have a large impact. Additionally, if non-synthetic sources are unable to fully replace synthetic nitrogen, there would likely be less feed available for livestock, and less manure available. We omit these second-order effects given the challenges in modeling them.
32. Approximately 5.5 billion acres, compared to Russia’s land area of 4.2 billion acres. https://data.mongabay.com/igapo/world_statistics_by_area.htm
33. This assumes that a 60% cut in nitrogen leads to a similar decrease in yields and that each new acre of farmland could provide the same amount of nitrogen as current farmland. In reality, yields would decrease slower than N applications in some areas (e.g. where N is currently overapplied) and faster in other areas (e.g. where there is little fertilizer applied). However, even if average global yields fall by 30% when N falls by 60%, billions more acres of cropland would be needed to maintain current production, holding all else constant.
34. Lassaletta et al. 2014 Supplemental Material 1 Methods Table 3
35. This is based on estimates of current average green manure fixation of 110 kgN/ha/yr, area planted with green manure crops of 24 million hectares and observations of potential clover fixation rates of ~220kgN/ha/yr. See excel workbook for calculations, assumptions and sources.
36. See excel workbook for calculations, assumptions and sources.
38. Poeplau, C., & Don, A. (2015). Carbon sequestration in agricultural soils via cultivation of cover crops—A meta-analysis. Agriculture, Ecosystems & Environment, 200, 33–41. https://doi.org/10.1016/j.agee.2014.10.024. P. 36.
39. Huber, V., Neher, I., Bodirsky, B. L., Höfner, K., & Schellnhuber, H. J. (2014). Will the world run out of land? A Kaya-type decomposition to study past trends of cropland expansion. Environmental Research Letters, 9(2), 024011. http://iopscience.iop.org/article/10.1088/1748-9326/9/2/024011 p.3, Fig 1.
40. Wu, W., You, L., & Chen, K. Z. (2015). Cropping Intensity Gaps: The Potential for Expanded Global Harvest Areas (No. 1459). Retrieved from https://papers.ssrn.com/sol3/papers.cfm?abstract_id=2685300. p. 21. Note: The study refers to cover crops, a term which is interchangeable with green manure in this case. Calculation: converted from ha to % using Wu et al's estimate of global cropland of 2.1 billion ha: 2,710,000 ha / 2,100,000,000 ha.
41. This assumes that the 13% of global cropland that can support an additional crop for harvest overlaps entirely with the area that could support a cover crop. This doesn’t explicitly account for residual N from leguminous fixation left after the first harvest. See excel workbook for calculations, assumptions and sources
42. Webb, J., Sørensen, P., Velthof, G., Amon, B., Pinto, M., Rodhe, L., ... & Reid, J. (2013). An assessment of the variation of manure nitrogen efficiency throughout Europe and an appraisal of means to increase manure-N efficiency. In Advances in agronomy (Vol. 119, pp. 371-442). Academic Press. p. 380-381 & 407-408
43. Such as applying manure to crops more often, storing it in modern systems, and incorporating it into soil shortly after applying it, rather than waiting or leaving it on the soil.
44. Oenema, O., & Tamminga, S. (n.d.). Nitrogen in global animal production and management options for improving nitrogen use efficiency. Science in China Series C: Life Sciences, 48(2), 871–887. https://doi.org/10.1007/bf03187126 p. 884
45. The contribution would likely be less or the land footprint of crop production would be greater. Since other sources cannot completely replace synthetic fertilizer, overall crop production would be lower and less feed would be available (or more land would be needed to maintain feed levels). This would likely reduce the amount of manure available. See excel workbook for calculations, assumptions and sources.
46. Bodirsky, B. L., Popp, A., Lotze-Campen, H., Dietrich, J. P., Rolinski, S., Weindl, I., … Stevanovic, M. (2014). Reactive nitrogen requirements to feed the world in 2050 and potential to mitigate nitrogen pollution. Nature Communications, 5(May), 3858. https://doi.org/10.1038/ncomms4858 p. 6 and Supplementary Data 1
47. Calculation: (66 TgN non-synthetic fertilizer current inputs + 36 TgN from additional nitrogen fixation + 20 TgN from manure improvements + 16.5 TgN from food waste & sewage improvements) / 163 TgN current inputs. See excel workbook for calculations, assumptions and sources.
48. See excel workbook for calculations, assumptions and sources.
49. Calculation based on assumption that average yield gap estimates apply globally
50. The lower bound estimates assumes 12% of land can add cover crops but cannot add harvest crops, and assumes a 20% yield gap.
The upper bound estimate assumes 0% of land can add cover crops but cannot add harvest crops, and assumes a 25% yield gap.
51. Assuming 10% of global cropland could add a cover crop/green manure, that the average green manure B fixation rate rose from 110 kgN/ha to 166 kgN/ha (representing a switch of some to clover for example), that 10 TgN of losses were eliminated from improving manure management and use, and that 25% of food waste and sewage N is recycled as fertilizer. See excel workbook for calculations, assumptions and sources.
52. https://www.nrcs.usda.gov/Internet/FSE_DOCUMENTS/nrcs143_012568.pdf
53. http://csanr.wsu.edu/can-manure-supply-n-and-p-to-ag/
54. Muller, A., Schader, C., El-Hage Scialabba, N., Brüggemann, J., Isensee, A., Erb, K.-H., … Niggli, U. (2017). Strategies for feeding the world more sustainably with organic agriculture. Nature Communications, 8(1), 1290. https://doi.org/10.1038/s41467-017-01410-w fig. 2